Throughout recent years, material researchers and physicists have been dealing with the planning of progressively refined materials for a large number of mechanical and logical applications. These materials incorporate manufactured polymers and hydrogels that could be presented inside the human body as a feature of clinical mediation.
Specialists at the Leibniz Foundation of Polymer Exploration Dresden, Technische Universität Dresden, and different organizations in Germany have as of late planned completely engineered materials with a powerful DNA-crosslinked framework that could demonstrate valuable for the making of organoids (fake organs) and other bio-mimetic frameworks. These materials, presented in Nature Nanotechnology, are adaptable, programmable, and moderately economical, making them worthwhile for clinical and organic exploration.
“Polymer science can make materials with great properties,” Elisha Krieg, one of the scientists who completed the review, told Phys.org. “Consider ordinary items like toys and bundling, yet additionally tactical armor carriers, parachutes, clinical inserts, and so on. In any case, these materials are exceptionally static—it isn’t difficult to change their properties; when broken, they can’t recuperate themselves; and their attributes are hard to foresee. Our gathering attempts to make materials that are much the same as living matter: versatile, self-mending, and customized to satisfy explicit capabilities.”
“Think of everyday items such as toys and packaging, as well as bullet-proof vests, parachutes, medical implants, and so on. However, these materials are exceedingly static—it is impossible to change their properties, they cannot heal themselves once broken, and their qualities are difficult to predict. Our research is working to create materials that are more like living matter: adaptable, self-healing, and designed to perform certain functions.”
Elisha Krieg, one of the researchers who carried out the study,
The field of DNA nanotechnology, first settled by Ned Seeman, centers around the design and assembly of counterfeit DNA structures with conceivable biomedical and biophysical applications. Past DNA nanotechnology studies demonstrated the way that DNA can be re-customized to control the properties of an issue at a nanometer scale.
The new work by Krieg and his associate Yu-Hsuan Peng expands on past examination endeavors in this field. Its goal was to make a delicate hydrogel framework that could have living cells and could, in this manner, be utilized to design tissue, organoids, clinical inserts, and other biophysical frameworks.
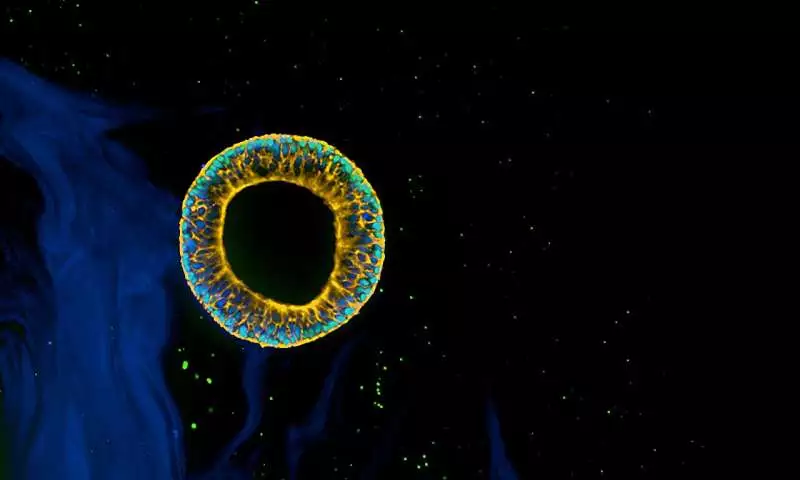
Human induced pluripotent stem cell cyst in DyNAtrix. Credit: Elisha Krieg and Yu-Hsuan Peng
“We trusted that by utilizing standards of DNA nanotechnology, we could definitively control the properties of our delicate material to ideally uphold cells and guide their turn of events,” Krieg made sense of. “Our objective was to make a material that is completely engineered, biocompatible, and—in particular—whose mechanical way of behaving ought to be flexible without definitely changing its substance creation. To wrap things up, it meant a lot to us for the material to be reasonable, as we trusted it would be applied by numerous different gatherings later on.”
To make a material that is flexible, engineered, biocompatible, programmable, and reasonable, the specialists blended two unique parts. The first are weighty, organically practical polymer chains.
“These polymer chains act as an underlying platform for the material,” Krieg said. “They have DNA side chains that permit further DNA-based modules to coordinate into the material, cross-connect the polymer, and supplement it with explicit capabilities.”
The second part of the group’s material includes remarkable DNA modules. These modules are connected to the material to program its properties and attributes, permitting it to act in unambiguous ways.
“One key advancement was our utilization of DNA-based libraries—complex combinations of DNA strands—which make cross-connecting exceptionally proficient,” Krieg said. “The successions of the DNA libraries likewise control significant qualities of the material, like the pliancy and solidness at various temperatures.”
The powerful DNA-crosslinked framework made by Krieg and his partners, named DyNAtrix, could be utilized to culture various cells in a research facility setting, including human pluripotent immature microorganisms and organoids. Surprisingly, their material is likewise self-recuperating and can undoubtedly be coordinated with 3D printing innovation to create various complex 3D tissues and designs.
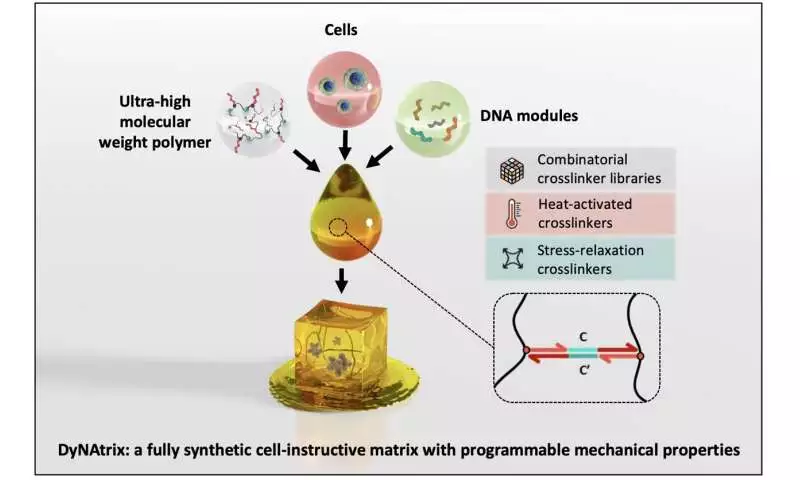
Credit: Elisha Krieg and Yu-Hsuan Peng
“Refined cells in DyNAtrix can assist with addressing inquiries in formative science; they very well may be utilized to culture tissues for regenerative medication or to test the impact of explicit medication up-and-comers with patient-determined cells. My expectation is that trying medications in an in-vitro cell culture framework will one day totally supplant creature testing.”
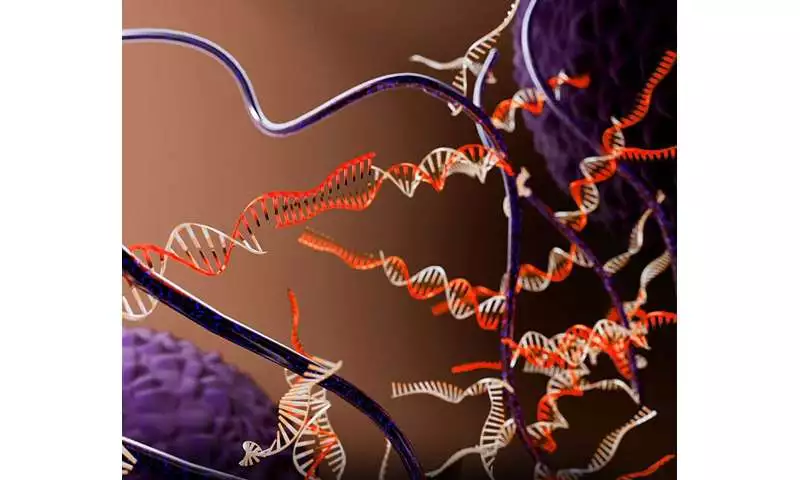
Artist impression of DNA crosslinked polymer network between cells. Credit: Elisha Krieg and Yu-Hsuan Peng.
The new material presented by this group of specialists can possibly be developed before long for biomechanical, biophysical, and biomedical examination. DyNAtrix is completely manufactured, programmable, simple to repeat for a huge scope, and can be changed with high accuracy. It could likewise be simpler to use in clinical settings compared with creature-determined materials like Matrigel (i.e., a framework separated from mouse growth cells that is often used to culture cells in the lab).
In their next examinations, Krieg, Peng, and their partners intend to additionally analyze the commonsense uses of their network. For example, they will begin teaming up with cell scholars, assisting them with utilizing DyNAtrix to handle explicit exploration issues.
“The customizable mechanical properties of DyNAtrix make it especially intriguing for responding to inquiries with regards to the area of mechanobiology; for example, how do mechanical qualities (firmness, consistency, and versatility) influence the improvement of cells? What importance do these impacts have for a living life form? What relevance do they have to sicknesses like disease? Furthermore, how precisely does the mechanical climate invigorate a reaction in living tissues?” Krieg added.
“Work in our lab is as of now centered around expanding the capacities of DyNAtrix. For example, by connecting fluorescent power sensors, we trust that mechanical collaborations among cells and their current circumstances can be evaluated through the magnifying lens.”
The DyNAtrix lattice is currently in its beginning phases of improvement, as it actually expects scientists to physically add DNA modules to prompt changes in a material’s properties. Later on, the group desires to further develop its creation and execution, for example, by utilizing more refined DNA-based response networks that would permit it to independently answer the way cells behave.
More information: Y.-H. Peng et al, Dynamic matrices with DNA-encoded viscoelasticity for cell and organoid culture, Nature Nanotechnology (2023). DOI: 10.1038/s41565-023-01483-3.