A method for “translating” quantum information between various quantum technologies has been discovered, which has significant implications for quantum computing, communication, and networking.
On Wednesday, the study was published in the Nature journal. It exemplifies an innovative method for converting quantum data from the format utilized by quantum computers to the format required for quantum communication.
Photons, which are light particles, are necessary for quantum information technologies; however, the frequencies at which these particles are used vary between technologies. For instance, superconducting qubits, such as those utilized by tech giants Google and IBM, are the foundation of some of the most prevalent quantum computing technology. Photons that move at microwave frequencies are used by these qubits to store quantum information.
But you can’t send microwave photons around to connect quantum computers or build a quantum network because their grip on their quantum information is too weak to last the trip.
“A lot of the technology that we use for traditional communication—cell phones, Wi-Fi, GPS, and so on—all employ microwave frequencies of light,”
Aishwarya Kumar, a postdoc at the James Franck Institute at University of Chicago.
Aishwarya Kumar, a postdoc at the James Franck Institute at the University of Chicago who is also the lead author of the paper, stated, “A lot of the technologies that we use for classical communication—cell phones, Wi-Fi, GPS, and things like that—all use microwave frequencies of light.” She is the lead author of the paper. Quantum communication, on the other hand, necessitates quantum information contained in a single photon, so you can’t do that. Additionally, thermal noise will obscure that information at microwave frequencies.”
The solution is to transfer the quantum information to an optical photon, a higher-frequency photon that is much more resistant to background noise. However, the data cannot be transferred directly from one photon to another; we require matter as an intermediary instead. While this is the goal of some experiments, Kumar’s experiment aimed for something more fundamental: atoms.
Atomic electrons can only ever have a limited number of energy levels. By striking an electron with a photon whose energy exactly matches the difference between the higher and lower levels, an electron can be excited to a higher energy level when it is at a lower energy level. Similarly, an atom emits a photon with the same energy as the energy difference between levels when an electron is forced to lower its energy level.
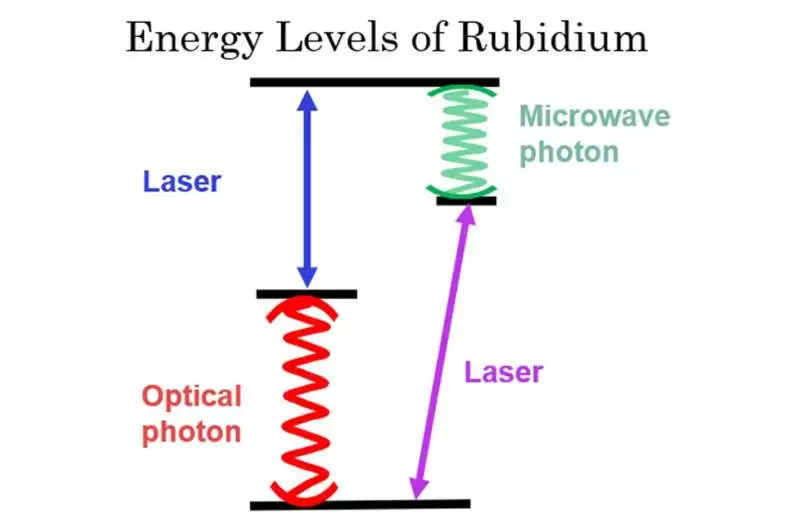
Rubidium’s electron energy levels are depicted in this diagram. Two of the energy level gaps correspond to optical and microwave photon frequencies, respectively. The electron is compelled to either ascend to higher levels or descend to lower levels by means of lasers. Credit: Aishwarya Kumar
The technology developed by Kumar makes use of two gaps in the levels of rubidium atoms: one whose energy is identical to that of an optical photon and one whose energy is identical to that of a microwave photon. The technology enables the atom to absorb a microwave photon containing quantum information and then emit an optical photon containing that quantum information by shifting the atom’s electron energies upward and downward using lasers. “Transduction” refers to the process of translating between various modes of quantum information.
The significant progress that scientists have made in manipulating such small objects makes it possible to use atoms effectively for this purpose. “In the last 20 or 30 years, we as a community have built remarkable technology that allows us to control essentially everything about the atoms,” Kumar stated. As a result, the experiment works well and is very controlled.”
He claims that the advancement of the field in cavity quantum electrodynamics, in which a photon is held in a superconducting, reflective chamber, is another factor in their success. The superconducting cavity strengthens the interaction between the photon and the matter that is contained within it by requiring the photon to move around within an enclosed space.
Their chamber actually looks more like a block of Swiss cheese than it does enclosed. However, what appear to be holes are actually tunnels that intersect in a very specific way, allowing photons or atoms to become trapped there. It’s a clever design that also lets researchers enter the chamber to inject atoms and photons.
The technology can be used either way: It is capable of transferring quantum information from optical photons to microwave photons and back again. As a result, it has the potential to be on either side of a long-distance connection between two superconducting qubit quantum computers and serve as a fundamental component of a quantum internet.
However, Kumar believes that this technology may have a lot more applications than just quantum networking. Its center capacity is to emphatically catch iotas and photons — a fundamental and troublesome errand in various quantum advancements across the field.
He stated, “The ability of this platform to generate really efficient entanglement is one of the things that we’re really excited about.” Ensnarement is key to nearly everything quantum that we care about, from figuring to reenactments to metrology and nuclear timekeepers. I can’t wait to see what else we can accomplish.”
More information: Aishwarya Kumar et al, Quantum-enabled millimetre wave to optical transduction using neutral atoms, Nature (2023). DOI: 10.1038/s41586-023-05740-2