Ultrashort pulses are typically produced by passively mode-locked lasers, but they can also be produced by optical parametric amplifiers (possibly with a super-continuum as an input) or free-electron lasers. In theory, longer pulses can be used to begin with and then compress, but in most cases, the input pulses for such compression are already ultrashort.
Researchers created a time-domain single-pixel imaging technique to accelerate the measurement of ultrafast pulses in the infrared and far-infrared wavelength ranges. When we look at something with our eyes or a camera, we can automatically gather enough pixels of light at visible wavelengths to create a clear image of what we see.
Light pulses generated by mode-locked lasers can be extremely short, especially when using passive mode-locking. There is no widely accepted definition of “ultrashort,” but it usually refers to pulses with pulse durations of a few tens of picoseconds or less, and often in the femtosecond range.
Scientists require far more sensitive tools to visualize a quantum object or phenomenon when the illumination is weak or coming from nonvisible infrared or far-infrared wavelengths. They have developed single-pixel imaging in the spatial domain, for example, as a method of packing and spatially structuring as many photons as possible onto a single-pixel detector and then creating an image using computational algorithms.
We can obtain a good hyperspectral image in a few seconds by combining our technique with single-pixel imaging in the spatial domain. That is much faster than previous attempts.
Jiapeng Zhao
Similarly, in the time domain, when an unknown ultrafast signal is either weak or in the infrared or far-infrared wavelengths, the ability of single-pixel imaging to visualize it is reduced. Based on the Spatio-temporal duality of light pulses, University of Rochester researchers developed a time-domain single-pixel imaging technique, described in Optica, that solves this problem by detecting 5 femtojoule ultrafast light pulses with a temporal sampling size of 16 femtoseconds.
This time-domain analogy of single-pixel imaging has the same advantages as its spatial counterparts: good measurement efficiency, high sensitivity, robustness against temporal distortions, and compatibility at multiple wavelengths.
There is no universally accepted definition of an ultrashort pulse. Typically, the term “ultrashort” refers to pulses lasting a few tens of femtoseconds, but in a broader sense, any pulse lasting less than a few picoseconds can be considered ultrashort. The distinction between “Ultrashort” and “Ultrafast” is necessary because the speed at which the pulse propagates is determined by the index of refraction of the medium through which it travels, whereas “Ultrashort” refers to the pulse wave packet’s temporal width.
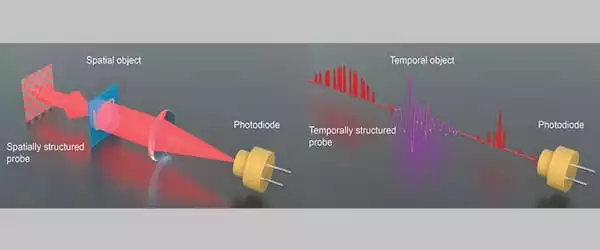
Lead author Jiapeng Zhao, a Ph.D. student in optics at the University of Rochester, says possible applications include a highly accurate spectrographic tool, demonstrated to achieve 97.5 percent accuracy in identifying samples using a convolutional neural network with this technique.
According to Zhao, who works in the Rochester research group of Robert Boyd, professor of optics, the technique can also be combined with single-pixel imaging to create a computational hyperspectral imaging system. The system can greatly accelerate image detection and analysis across a wide range of frequency bands. This could be especially useful in medical applications, where detection of nonvisible light emitted by human tissue at various wavelengths can indicate disorders such as high blood pressure.
“We can obtain a good hyperspectral image in a few seconds by combining our technique with single-pixel imaging in the spatial domain. That is much faster than previous attempts” Zhao claims.
When a pre-characterized reference pulse is available, spectral interferometry (SI) is a linear technique that can be used. It specifies the intensity as well as the phase. The algorithm used to extract the intensity and phase of the SI signal is straightforward. SPIDER is a nonlinear self-referencing technique based on spectral shearing interferometry.
The method is similar to SI, except that the reference pulse is a spectrally shifted replica of itself, allowing the spectral intensity and phase of the probe pulse to be obtained using a direct FFT filtering routine similar to SI, but which requires integration of the phase extracted from the interferogram to obtain the probe pulse phase.
Boyd and Xi-Cheng Zhang from Rochester University, Jianming Dai from Tianjin University, and Boris Braverman from the University of Ottawa are also coauthors. The Office of Naval Research, the National Natural Science Foundation of China, and the National Key Research and Development Program of China all provided funding for this project.