As the globe strives to enhance the efficiency of cars and other vehicles in order to reduce greenhouse gas emissions and increase the range of electric vehicles, researchers are looking for ever lighter materials that are sturdy enough to be utilized in car bodywork.
Not only is the new carbon fiber inexpensive to produce, but it also has advantages over previous carbon fiber materials in that it has compressional strength, allowing it to be employed in load-bearing applications. The new method is reported in a paper published in the journal Science Advances by graduate student Asmita Jana, research scientist Nicola Ferralis, professor Jeffrey Grossman, and five others from MIT, the Western Research Institute in Wyoming, and Oak Ridge National Laboratory in Tennessee.
The study began four years ago in response to a request from the Department of Energy, which was looking for ways to make cars more efficient and cut fuel use by reducing their overall weight. If you compare the same model car today to the same model car 30 years ago, it’s much heavier, “Ferraris explains. Within the same category, the weight of cars has increased by more than 15%.
Because a heavier automobile necessitates a larger engine, stronger brakes, and so on, reducing the weight of the body or other components has a knock-on effect that results in more weight savings. The DOE is pushing for the development of lightweight structural materials that are as safe as today’s traditional steel panels but can be produced at a low enough cost to potentially replace steel entirely in regular automobiles.
Composites comprised of carbon fibers are not a novel concept, but they have only been used in a few high-end vehicles thus far. The new study hopes to change that by providing a low-cost starting material and relatively easy processing methods.
Carbon fibers of the quality required for automotive applications presently cost at least $10 to $12 per pound, according to Ferralis, and “may be a lot more,” up to hundreds of dollars per pound for specialist applications such as spacecraft components. This contrasts to around 75 cents per pound for steel or $2 for aluminum, although these prices fluctuate greatly and the materials are frequently sourced from outside. At that price, he claims that building a pickup truck out of carbon fiber rather than steel would cost nearly twice as much.
These fibers are often manufactured from petroleum-derived polymers (such as polyacrilonitrile), but with a costly intermediary step of polymerizing the carbon components. According to Ferralis, the cost of the polymer can account for more than 60% of the overall cost of the final fiber. Instead of starting with a refined and processed petroleum product, the team’s innovative strategy employs petroleum pitch, which is essentially the dregs left over from the refining process. It’s sometimes referred to as the “bottom of the barrel,” Ferralis explains.
“The pitch is quite messy,” he admits. It’s a jumble of mixed heavy hydrocarbons, and “in a way, that’s what makes it wonderful, in a way, because there’s so much chemistry that can be exploited.” That makes it a fascinating material to start with. “
It’s ineffective for combustion because, while it can burn, it’s too polluting a fuel to be viable, especially in light of stricter environmental rules. Because there is so much of it, he continues, “the inherent worth of these things is quite poor, thus it is frequently landfilled.” Coal pitch, a similar material that is a byproduct of coking coal and is used, for example, in steel production, was also evaluated as an alternate source of pitch by the researchers. This technique produces roughly 80% coke and 20% coal pitch, “which is really a waste,” he explains.
Working with Oak Ridge National Laboratory researchers who had expertise in manufacturing carbon fibers under a variety of conditions ranging from lab scale to pilot plant scale, the team set about determining ways to predict performance in order to guide the choice of conditions for those fabrication experiments.
“The new route we’re developing is not just a cost effect, It might open up new applications, and it doesn’t have to be vehicles.”
Ferralis says.
“The procedure that you need to actually generate a carbon fiber [from pitch] is actually incredibly small, both in terms of energy requirements and the actual processing that you need to conduct,” added Ferralis.
Jana notes that pitch is “composed of these heterogeneous groups of molecules, where you would anticipate the qualities to shift substantially if you change the form or size,” whereas an industrial material must have highly consistent properties.
By meticulously modeling the ways bonds form and crosslink between the constituent molecules, Jana was able to establish a method of anticipating how a given set of processing settings would affect the resultant fiber qualities. “We were able to duplicate the data with such stunning accuracy,” she says, “to the point where companies could take those graphs and forecast” features like fiber density and elastic modulus.
The research showed that by altering the beginning conditions, carbon fibers could be created that were not only strong in tension, as most such fibers are, but also strong in compression, implying that they may potentially be employed in load-bearing applications. This opens up totally new avenues for the application of these materials.
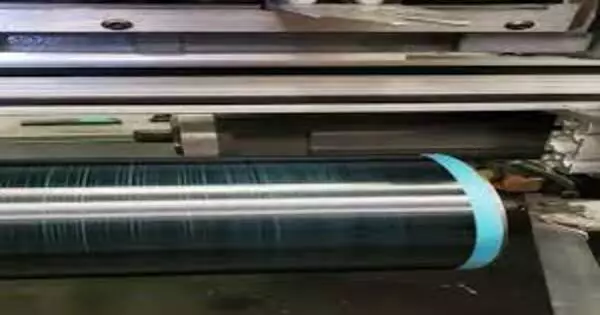
The DOE’s request was for projects to reduce the cost of lightweight materials to less than $5 per pound, but the MIT team reckons that their technology can do better, reaching around $3 per pound, though they haven’t yet done a comprehensive economic study.
“The new path we’re constructing isn’t only a cost-cutting measure,” Ferralis explains. “It might lead to other uses, and they don’t have to be automobiles.” The fact that the fibers must be formed into a cloth and spread out in exact, detailed patterns adds to the complexity of producing conventional fiber composites. “It’s to compensate for the lack of compressive strength,” he explains. It takes engineering to overcome the material’s shortcomings, he says, but with the new technique, none of that extra complexity is required.
Taishan Zhu and Yanming Wang from MIT, Jeramie Adams from Western Reserve University, and Logan Kearney and Amit Naskar from Oak Ridge National Laboratory were part of the research team. The work was supported by the U.S. Department of Energy.
The source of the story:
The materials have been given by the Massachusetts Institute of Technology. David L. Chandler wrote the original. Please keep in mind that content may be altered for style and length.