Researchers devised a method that allows quantum sensors to detect any arbitrary frequency while maintaining their ability to measure nanometer-scale features. Quantum sensors detect the most minute variations in magnetic or electrical fields, but they have only been capable of detecting a few specific frequencies, limiting their utility.
Quantum sensors, which detect the smallest variations in magnetic or electrical fields, have enabled precision measurements in materials science and fundamental physics. However, these sensors have only been capable of detecting a few specific frequencies of these fields, limiting their usefulness. MIT researchers have now developed a method that allows such sensors to detect any arbitrary frequency while maintaining their ability to measure nanometer-scale features.
The new method, for which the team has already applied for patent protection, is described in the journal Physical Review X, in a paper by graduate student Guoqing Wang, professor of nuclear science and engineering and of physics Paola Cappellaro, and four others at MIT and Lincoln Laboratory.
Quantum sensors can take many forms; they are essentially systems in which some particles are in such a delicately balanced state that even minor variations in the fields they are exposed to affect them. These can take the form of neutral atoms, trapped ions, or solid-state spins, and research into such sensors has grown rapidly. For example, physicists use them to investigate exotic states of matter such as time crystals and topological phases, whereas other researchers use them to characterize practical devices such as experimental quantum memory or computation devices. However, many other phenomena of interest span a much wider frequency range than today’s quantum sensors can detect.
The system may open up new applications in biomedical fields because it can access a range of frequencies of electrical or magnetic activity at the level of a single cell. It would be extremely difficult to obtain useful resolution of such signals using current quantum sensing systems.
Paola Cappellaro
The team’s new system, dubbed a quantum mixer, uses a microwave beam to inject a second frequency into the detector. This converts the frequency of the field being studied into a different frequency (the difference between the original frequency and that of the added signal) that is tuned to the specific frequency to which the detector is most sensitive. This simple procedure allows the detector to zero in on any desired frequency while preserving the sensor’s nanoscale spatial resolution.
In their experiments, the team used a specific device based on an array of nitrogen-vacancy centers in diamond, a widely used quantum sensing system, and successfully demonstrated detection of a signal with a frequency of 150 megahertz using a qubit detector with a frequency of 2.2 gigahertz – a detection that would be impossible without the quantum multiplexer. They then conducted detailed analyses of the process by developing a theoretical framework based on Floquet theory and testing the numerical predictions of that theory in a series of experiments.
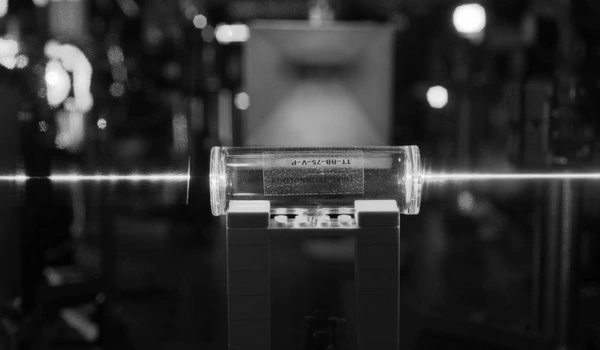
While their tests used this specific system, Wang says, “the same principle can be also applied to any kind of sensors or quantum devices.” The system would be self-contained, with the detector and the source of the second frequency all packaged in a single device.
Wang says that this system could be used, for example, to characterize in detail the performance of a microwave antenna. “It can characterize the distribution of the field [generated by the antenna] with nanoscale resolution, so it’s very promising in that direction,” he says.
Other methods of modifying the frequency sensitivity of some quantum sensors exist, but they require the use of large devices and strong magnetic fields, which blur out fine details and make it impossible to achieve the very high resolution that the new system provides. Today, Wang says, “you need to use a strong magnetic field to tune the sensor, but that magnetic field can potentially break the quantum material properties, which can influence the phenomena that you want to measure.”
According to Cappellaro, the system may open up new applications in biomedical fields because it can access a range of frequencies of electrical or magnetic activity at the level of a single cell. According to her, it would be extremely difficult to obtain useful resolution of such signals using current quantum sensing systems. This system may be able to detect output signals from a single neuron in response to a stimulus, for example, which typically include a lot of noise, making such signals difficult to isolate.
The system could also be used to characterize the behavior of exotic materials, such as 2D materials, which are being studied extensively for their electromagnetic, optical, and physical properties.
In ongoing research, the team is looking into ways to expand the system so that it can probe a wide range of frequencies at once, rather than the current system’s single frequency targeting. They will also continue to define the system’s capabilities using more powerful quantum sensing devices at Lincoln Laboratory, where some of the research team is based.