Graphene quantum dots (GQDs) are small pieces of graphene, which are typically less than 10 nanometers in size. Due to their unique properties, GQDs have been proposed as a potential candidate for various applications, including magnetic field sensing. GQDs have a high surface-to-volume ratio and are sensitive to changes in their local environment, which makes them ideal for sensing applications. When exposed to a magnetic field, GQDs can exhibit a change in their electronic and optical properties, which can be detected and measured.
According to a new study, trapped electrons traveling in circular loops at high speeds inside graphene quantum dots are highly sensitive to external magnetic fields and could be used as novel magnetic field sensors with unique capabilities.
Electrons in graphene (an atomically thin form of carbon) behave as if they have no mass, similar to photons, which are massless light particles. Despite the fact that graphene electrons do not travel at the speed of light, they have the same energy-momentum relationship as photons and are thus “ultra-relativistic.” When these electrons are trapped inside a quantum dot, they travel at high speeds in circular loops around the dot’s edge.
“These current loops create magnetic moments that are very sensitive to external magnetic fields,” explained Jairo Velasco Jr., associate professor of physics at UC Santa Cruz. “The sensitivity of these current loops stems from the fact that graphene electrons are ultra-relativistic and travel at high velocity.”
These current loops create magnetic moments that are very sensitive to external magnetic fields. The sensitivity of these current loops stems from the fact that graphene electrons are ultra-relativistic and travel at high velocity.
Jairo Velasco Jr.
Velasco is a co-author on a paper about the new findings that was published in Nature Nanotechnology. His team at UC Santa Cruz created and studied quantum dots in graphene using a scanning tunneling microscope (STM). Scientists from the University of Manchester in the United Kingdom and the National Institute of Materials Science in Japan are among his project collaborators.
“It was a very collaborative effort,” Velasco said. “We did the measurements at UCSC in my lab, and then we worked closely with theoretical physicists at the University of Manchester to understand and interpret our data.”
The unique optical and electrical properties of quantum dots, which are frequently made of semiconductor nanocrystals, are due to electrons being confined within a nanoscale structure, governing their behavior. Because the resulting electronic structure is similar to that of atoms, quantum dots are frequently referred to as “artificial atoms.” Velasco’s method produces quantum dots in various forms of graphene by using an electrostatic “corral” to confine the speeding electrons of graphene.
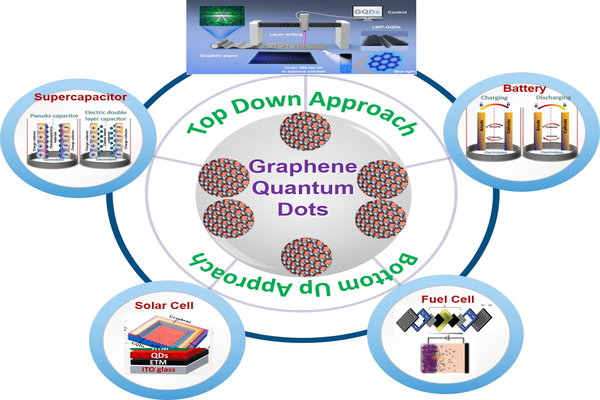
“Part of what makes this interesting is the fundamental physics of this system, as well as the opportunity to study atomic physics in the ultra-relativistic regime,” he explained. “At the same time, this has intriguing potential applications as a new type of quantum sensor that can detect magnetic fields at the nano scale with high spatial resolution.”
According to co-first author Zhehao Ge, a UCSC graduate student in physics, additional applications are possible. “Our findings also suggest that graphene quantum dots can potentially host a giant persistent current (a perpetual electric current that does not require an external power source) in a small magnetic field,” Ge said. “This type of current has the potential to be used for quantum simulation and quantum computation.”
The researchers investigated quantum dots in both monolayer graphene and twisted bilayer graphene. The graphene is supported by an insulating layer of hexagonal boron nitride, and applying a voltage with the STM tip generates charges in the boron nitride, which serve to electrostatically confine electrons in the graphene.
Despite the fact that Velasco’s lab uses STM to create and study graphene quantum dots, a simpler system based on metal electrodes in a cross-bar array could be used in a magnetic sensor device. Due to the high flexibility of graphene, the sensor could be integrated with flexible substrates to enable magnetic field sensing of curved objects.
“You could have many quantum dots in an array,” Velasco explained, “and this could be used to measure magnetic fields in living organisms or to understand how the magnetic field is distributed in a material or a device.”