The College of Engineering at Oregon State University discovered a means to boost the efficiency of a sort of grid-scale storage that is critical for a global transition to renewable energy. Moving toward net-zero carbon emissions requires coping with the intermittent, unpredictable nature of renewable energy sources like wind and solar, as well as addressing supply and demand mismatches, according to OSU’s Nick AuYeung and Ph.D. student Fuqiong Lei, who led the study.
These issues, according to AuYeung, need energy storage methods other than pumped hydro plants, which contain a turbine between two water reservoirs at varying heights, and massive lithium-ion batteries.
The computer modeling study led by AuYeung, associate professor of chemical engineering, and Lei discovered that compressed air, one of the supplementary energy storage solutions, might be improved through chemical processes. Reversible reactions have the ability to absorb energy in the form of heat and then conserve energy that would otherwise be lost. According to AuYeung, the findings, which were published in Energy Conversion and Management, are also applicable to a related technique, liquid air energy storage.
The liquid and compressed air approaches, as their names suggest, harness energy that can be accessed when needed by allowing stored air – either pressured or cooled to a liquid state – to expand and pass through electricity-generating turbines.
We looked at TCES with packed beds that were full of rocks and barium oxides. Because of the comparatively low heat capacity and heat of reaction for the barium oxides, our results demonstrated a similar round-trip efficiency across beds with TCES and beds without.
AuYeung
However, both CAES (compressed air energy storage) and LAES (liquid air) perform rather poorly in a category known as round-trip efficiency, according to AuYeung. With either, only roughly half of the energy put in can be extracted; imagine making a $1,000 bank deposit but only have $500 available for withdrawal due to various expenses.
“One advantage of CAES is that it allows energy to be stored at vast sizes, which is a challenge for electrochemical battery technologies,” he explained. “However, achieving high round-trip efficiency is a significant issue for typical CAES.”
According to AuYeung, in a traditional CAES process, electricity is used to compress air, and the compressed air is kept below ground in a cavern or a pressure vessel. When air is compressed, its temperature rises; nevertheless, this heat is often viewed as waste, and so goes unrecovered and unusable.
“To discharge the air to produce power, it’s commonly heated with natural gas to improve the enthalpy, or total system energy, of the turbine feed,” he explained. “When heat lost during storage is taken into account, the overall round-trip efficiency — the ratio of turbine output work to work used through compression — is only between 40% and 50%.”
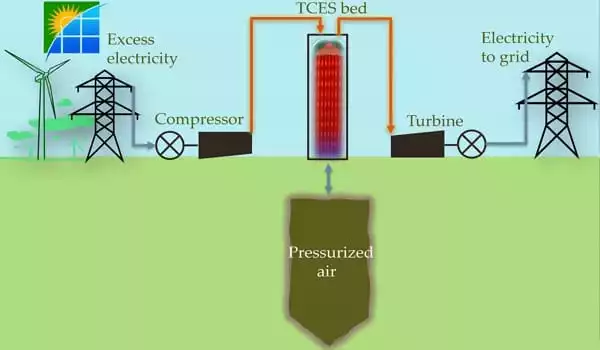
AuYeung and colleagues from Oklahoma State University, Mississippi State University, and Michigan State University devised a storage system to improve efficiency (thermochemically recovering lost heat), as well as a mathematical model for its design and operation. He claims that one advantage of thermochemical energy storage, or TCES, over other systems is a higher energy density enabled by collecting heat in the form of chemical bonds.
The researchers used their model to examine the efficacy of TCES implemented into thermal energy storage via “packed beds” — vessels filled with some kind of solid packing medium, where energy is transferred to the solid via a heat transfer fluid such as air. Alumina, ceramic, or crushed rock are common filler materials. Packed beds are considered “sensible” storage since energy is captured as the filler material changes temperature.
“We looked at TCES with packed beds that were full of rocks and barium oxides,” AuYeung explained. “Because of the comparatively low heat capacity and heat of reaction for the barium oxides, our results demonstrated a similar round-trip efficiency across beds with TCES and beds without. With a 20-hour storage duration after charging, we achieved 60 percent round-trip efficiency for both systems. Other types of thermal storage cannot hold heat for lengthy periods of time because they cool.”
Importantly, he highlighted that with TCES material placed above the packed beds, the turbine air inlet temperature was more stable — higher for longer — which is critical to optimal power generation and thus desirable to utilities. Furthermore, AuYeung stated that the model indicates that with future upgraded materials, round-trip efficiency and storage time could improve.
“We came up with a hypothetical material with the same heat capacity as rocks but a thermochemical storage capacity three times that of barium oxides, and we looked at that hypothetical material in our model to better highlight the potential of the concept,” he added. “The results demonstrated that a possible round-trip efficiency improvement of more than 5%, as well as extended storage durations, can be reached. Furthermore, 45 percent less filler volume would be required to reach storage capacity comparable to rock-fill “d” beds.”
According to AuYeung, the barium chemistry on which the initial model was built was the first thing that sprang to mind for the researchers, but it has a disadvantage in that its heat capacity is quite low. “There are non-oxygen chemistries like hydrates and carbonates that have the potential qualities we looked at — high heat capacity, high heat of reaction — but for now we haven’t identified one for a redox material that runs on oxygen swing,” he added. “Perhaps the next step for us or others with more material expertise would be to try to identify new materials.”