Another computational examination by scholars at the U.S. Department of Energy’s Brookhaven Public Lab and Wayne State College upholds the possibility that photons (a.k.a. particles of light) slamming into weighty particles can make a liquid of “firmly connected” particles. In a paper just distributed in Actual Survey Letters, they show that estimations depicting such a framework coordinate with information gathered by the Map Book Finder at Europe’s Huge Hadron Collider (LHC).
As the paper makes sense of, the estimations depend on the hydrodynamic molecule stream found in head-on crashes of different kinds of particles at both the LHC and the Relativistic Heavy Particle Collider (RHIC), a DOE Office of Science client office for atomic and physical science research at Brookhaven Lab. These estimates also depict the stream designs seen in close miss impacts, where photons that form a cloud around the speeding particles collide with particles in the opposite bar, with only minor changes.
“The end result is that utilizing similar systems we use to depict lead and proton-lead crashes, we can portray the information of these super fringe impacts where we have a photon slamming into a lead core,” said Brookhaven Lab scholar Bjoern Schenke, a co-creator of the paper. “That lets you know it’s plausible that in these photon-particle impacts, we make a little thick, firmly connecting medium that is very much depicted by hydrodynamics—very much like in the bigger frameworks.”
“The conclusion is that by utilizing the same framework to describe lead-lead and proton-lead collisions, we can characterize the data from these ultra-peripheral collisions in which a photon strikes a lead nucleus.”
Brookhaven Lab theorist Bjoern Schenke, a co-author of the paper.
Fluid signatures
Perceptions of particles streaming in trademark ways have been key proof that the bigger crash frameworks (lead and proton-lead impacts at the LHC and gold and proton-gold crashes at the RHIC) make an almost wonderful liquid. The stream designs were remembered as resulting from the enormous strain angles created by the massive number of firmly connecting particles created where the impacting particles crossed over.
“By crushing these high-energy cores together, we’re making such a high-energy thickness—ppacking the motor energy of these folks into such a little space—tthat this stuff basically acts like a liquid,” Schenke said.
Round particles (counting protons and cores) impacting head-on are supposed to create a uniform strain slope. However, partially covering crashes generate an oval, almond-shaped pressure slope that pushes more high-energy particles out along the short hub than the opposite side.
This “elliptic stream” design was perhaps the earliest clue that molecule crashes at RHIC could make a quark-gluon plasma, or QGP—aa hot soup of the key structure obstructs that make the protons and neutrons of cores and particles. Researchers were at first amazed by the QGP’s fluid-like way of behaving. Yet, they later settled on the elliptic stream as a characterizing element of QGP and proof that the quarks and gluons were all the while connecting firmly, in any event, when liberated from control inside individual protons and neutrons. Later perceptions of similar stream designs in the impacts of protons with large cores suggest that these proton-core crash frameworks can also produce small amounts of quark-gluon soup.
“Our new paper is tied in with pushing this to much further limits, taking a gander at impacts among photons and cores,” Schenke said.
Changing the shot
It has for some time been known that those super fringe crashes could make photon-core connections, involving the actual cores as the wellspring of the photons. That is on the grounds that charged particles advanced to high energies, similar to the lead cores and particles advanced at the LHC (and gold particles at the RHIC), emanate electromagnetic waves—particles of light. Thus, each sped-up lead particle at the LHC is basically encircled by a haze of photons.
“At the point when two of these particles pass each other intently without impacting, you can consider one radiating a photon, which then stirs things up around town with the other particle going the alternate way,” Schenke said. “Those occasions happen a ton; it’s simpler for the particles to scarcely miss each other than to exactly hit each other.”
Map-book researchers have as of late distributed information on charming stream-like signs from these photon-core crashes.
“We needed to set up exceptional data collection methods to select these novel impacts,” said Blair Seidlitz, a Columbia College physicist who helped set up the Map book and setting off framework for the examination while an alumni understudy at the University of Colorado, Boulder.”Subsequent to gathering an adequate amount of information, we were shocked to find stream-like signals that were like those seen in lead and proton-lead crashes, despite the fact that they were somewhat more modest.”
Schenke and his partners set off to see whether their hypothetical estimations could precisely depict the molecule stream designs.
They utilized the very hydrodynamic estimations that depict the behavior of particles created in lead and proton-lead crash frameworks. Yet, they made a couple of adjustments to represent the “shot” striking the lead core transforming from a proton to a photon.
As per the laws of material science (explicitly, quantum electrodynamics), a photon can go through quantum changes to turn into one more molecule with similar quantum numbers. A rho meson, a molecule made of a specific mix of quarks and antiquarks kept intact by gluons, is one of the most probable consequences of those photon changes.
In the event that you recall the proton—mmade of three quarks—tthis two-quark Rho molecule is only a stage down the complexity stepping stool.
“Rather than having a gluon dispersion around three quarks inside a proton, we have the two quarks (quark and antiquark) with a gluon conveyance around those to slam into the core,” Schenke said.
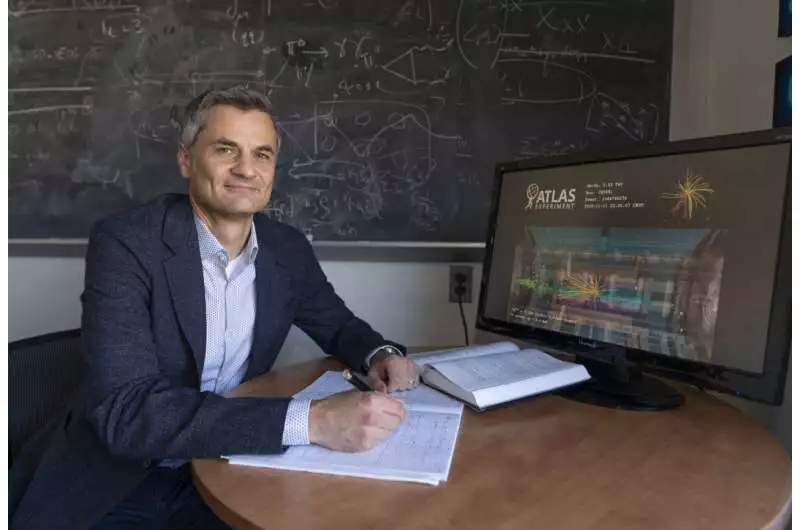
Brookhaven Lab scholar Bjoern Schenke’s hydrodynamic estimations coordinate with information from crashes of photons with nuclear cores at the Huge Hadron Collider’s map book finder, proposing those impacts make a liquid of “firmly connecting” particles.
Representing energy
The estimates also had to account for the large difference in energy in these photon-core crash frameworks when compared to proton-lead and, in particular, lead.
“The produced photon that is slamming into the lead will not convey the entire force of the lead core from which it came, but only a small part of that.”As a result, the crash energy will be significantly reduced,” Schenke explained.
That energy contrast ended up being much more significant than the difference in shots.
In the most fiery lead or gold-weighted particle impacts, the example of particles arising in the plane crossing over to the impacting surface endures by and large regardless of how far you look from the crash point along the beamline (in the longitudinal course). Yet, when Schenke and partners displayed the examples of particles expected to rise out of lower-energy photon-lead crashes, it became clear that including the 3D subtleties of the longitudinal course had an effect. The model showed that the math of the molecule dispersions changes quickly with expanding longitudinal distance; the particles become “decorrelated.”
“Depending on their longitudinal position, the particles see different strain slopes,” Schenke explained.
“Thus, for these low-energy photon-lead impacts, it is vital to run a full 3D hydrodynamic model (which is more computationally demanding) on the grounds that the molecule conveyance changes more quickly as you head out in the longitudinal path,” he said.
At the point when the scholars analyzed their expectations utilizing this lower-energy, full 3D hydrodynamic model with the molecule stream designs seen in photon-lead crashes by the Map Book Finder, the information and hypotheses matched up pleasantly, basically for the clearest elliptic stream design, Schenke said.
Suggestions and what’s to come
“From this outcome, it seems as though it’s possible that even in photon-weighty particle crashes, we have a firmly connecting liquid that answers the underlying impact math, as depicted by hydrodynamics,” Schenke said. “In the event that the energies and temperatures are sufficiently high,” he added, “there will be a quark-gluon plasma.”
Seidlitz, the Map book physicist, remarked, “It was extremely fascinating to see these outcomes proposing the development of a little drop of quark-gluon plasma, as well as how this hypothetical examination offers substantial clarifications regarding why the stream marks are a bit more modest in photon-lead impacts.”
Extra data gathered by Map Book and other tests at RHIC and the LHC over the next few years will enable more precise examinations of particles moving from photon-core crashes.These tests will help distinguish the hydrodynamic estimation from another possible explanation, where the stream designs are not a result of the framework’s reaction to the underlying math.
In the long run, tests at an Electron-Particle Collider (EIC), which an office wanted to replace RHIC at Brookhaven Lab at some point in the next ten years, could provide more conclusive results.
More information: Wenbin Zhao et al, Collectivity in Ultraperipheral Pb + Pb Collisions at the Large Hadron Collider, Physical Review Letters (2022). DOI: 10.1103/PhysRevLett.129.252302. journals.aps.org/prl/abstract/ … ysRevLett.129.252302
Journal information: Physical Review Letters