A recent study conducted by the University of Virginia appears to challenge a long-held understanding of how associative polymers, a class of materials with unique self-healing and flow properties, function at the molecular level.
The study’s lead author, UVA assistant professor of materials science and engineering and chemical engineering Liheng Cai, stated that the new discovery has significant repercussions for the numerous everyday applications of these materials, such as the engineering of recyclable plastics and human tissue and the control of paint’s consistency to prevent drips.
New associative polymers created in Cai’s lab at the UVA School of Engineering and Applied Science by postdoctoral researcher Shifeng Nian and Ph.D. student Myoeum Kim enabled the discovery, which was published in the journal Physical Review Letters. Before Cai joined UVA in 2018, he had co-developed a theory that led to the breakthrough.
“This necessitates careful control over the local environment, such as polymer temperature and humidity. My lab has built a set of methodologies and mechanisms for doing so throughout the years.”
Shiwang Cheng, an assistant professor in Michigan State University’s chemical engineering.
“Shifeng and Myoeum basically made a clever exploratory stage to concentrate on the elements of cooperative polymers in ways that were unimaginable previously,” Cai said.
“This provided us with a fresh perspective on the behavior of the polymers and presents opportunities to enhance our comprehension of particularly difficult polymer science research areas. In addition, the research aids in the creation of customized self-healing materials from a technological point of view.”
Monomers, or repeating units, make up polymers, which are macromolecules. Scientists can create polymeric materials with specific properties by rearranging or combining these units and tinkering with their bonds.
Polymers can also change states depending on temperature and force—for example, pushing a solid gel through a hypodermic needle—from hard and rigid to rubbery or even fluid.
Particularly unique are associative polymers. Their moieties — a general term for sub-atomic subunits with adjustable actual properties — are kept intact by reversible bonds, meaning they can fall to pieces and yet again lose structure.
Inaccessible to conventional polymers, this procedure makes it possible to access macroscopic properties. As a result, associative polymers offer answers to some of the most pressing issues pertaining to health and sustainability. For instance, associative polymers are utilized to engineer biomaterials with physical properties essential to tissue engineering and regeneration, to create tough, self-healing polymers, and as viscosity modifiers in fuels.
One key to the UVA cooperation was defeating a material component that has hindered scientists for quite a long time. Scientists work in the laboratory with materials whose bonds can break and re-form at “laboratory time scales,” which refer to the time frames that they can observe through experiments. Notwithstanding, in virtually all current trial frameworks, the moieties total into little groups, which prevents an exact investigation of the connection between reversible bonds and polymer conduct.
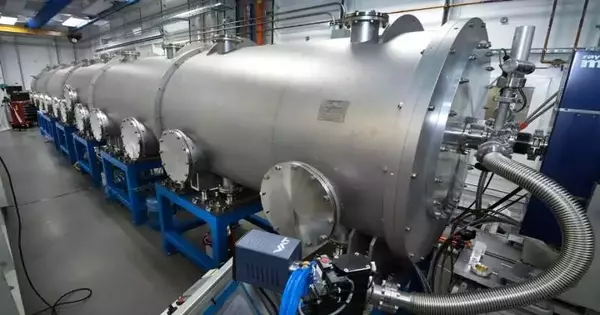
The soft matter interface beamline, which can be seen here, was used by Cai’s collaborators at the National Synchrotron Light Source II in their experiments to reveal the inner makeup of the polymers without harming the samples. Credit: University of Virginia.
Cai’s group created novel associative polymers with linkages that are equally dispersed throughout the material and have a wide range of densities. The researchers worked with Mikhail Zhernenkov, a scientist at the US Department of Energy’s Brookhaven National Laboratory, to prove that their materials do not form clusters. They carried out studies at the National Synchrotron Light Source II with a powerful X-ray tool—the soft matter interactions beamline—to expose the underlying makeup of the polymers without destroying the samples.
Cai’s group was able to precisely examine the effects of reversible interactions on the dynamics of associative polymers thanks to these brand-new associative polymers.
The characteristics of a material’s viscosity—how freely it flows—and elasticity—its capacity to snap back after being deformed—are all examples of dynamics and behavior. For example, the temperature at which molecule movement slows down to a rigid, “glassy” state is an example of dynamics and behavior. When designing a biomaterial that is compatible with human tissue and can self-renew after being injected, for instance, a combination of these characteristics is frequently desired.
For a very long time, it had been acknowledged that when the reversible bonds stay in salvageable shape, they go about as crosslinkers, bringing about a rubbery material. However, the UVA-led team discovered otherwise.
The team precisely measured the flow behavior of their polymers over a wide range of time scales in collaboration with Shiwang Cheng, an expert in flow dynamics and assistant professor in the chemical engineering and materials science department at Michigan State University.
Cheng stated, “This requires careful control over the local environment, such as the polymers’ temperature and humidity.” My lab has developed a set of procedures and systems for doing so over time.”
The team discovered that the bonds can dissipate energy and slow down the movement of polymers without forming a rubbery network. Suddenly, the examination showed that reversible connections impact the polymers’ smooth characteristics as opposed to their viscoelastic reach.
Cai stated, “Our associative polymers provide a system that allows for the independent investigation of the effects of reversible interactions on [polymer] movement and glassy behavior.” This might give us a chance to learn more about the difficult physics of glassy polymers like plastics.”
From their examinations, Cai’s group likewise fostered another atomic hypothesis that makes sense of the way familiar polymers behave, which could lead to pondering how to design them with upgraded properties like high solidness and fast self-recovery.
In addition to Nian, Kim, Cheng, and Zhernenkov, Cai worked with Ting Ge, an expert in computational simulations who is also an assistant professor of chemistry and biochemistry at the University of South Carolina. Quan Chen, who works at the State Key Lab of Polymer Physics and Chemistry at the Changchun Institute of Applied Chemistry and provided the initial code for analyzing the flow behavior of polymers, also contributed to the project.
The paper, “Elements of Affiliated Polymers with High Thickness of Reversible Bonds,” shows up in the June 2 issue of Actual Survey Letters and is included as an Editors’ Idea.
More information: Shifeng Nian et al, Dynamics of Associative Polymers with High Density of Reversible Bonds, Physical Review Letters (2023). DOI: 10.1103/PhysRevLett.130.228101
Evgeny B. Stukalin et al, Self-Healing of Unentangled Polymer Networks with Reversible Bonds, Macromolecules (2013). DOI: 10.1021/ma401111n