Envision a street with two paths leading to every house. One path is for slow vehicles, and the other is for quick ones. For electrons moving along a quantum wire, scientists in Cambridge and Frankfurt have found that there are likewise two “paths,” yet electrons can take both simultaneously!
Current in a wire is conveyed by the progression of electrons. At the point when the wire is extremely limited (one-layered, 1D), at that point, electrons can’t surpass one another, as they firmly repulse one another. Current, or energy, is conveyed by rushes of pressure as one molecule pushes on the next.
“It’s as if the automobiles (like charges) are moving in the slow lane, but their passengers (like spins) are traveling faster, in the fast lane, Even if the cars and people slow down or accelerate, they remain apart!”
Pedro Vianez, who conducted the observations for his Ph.D. at Cambridge’s Cavendish Laboratory.
It has for some time been realized that there are two kinds of excitation for electrons, as notwithstanding their charge, they have a property called turn. Twist and charge excitations travel at fixed but various rates, as anticipated by the Tomonaga-Luttinger model ages ago. In any case, scholars can’t compute what definitively occurs past just a little bother, as the connections are excessively mind-boggling. The Cambridge group has estimated these rates as their energies are shifted, and found that an exceptionally straightforward picture arises (presently distributed in the diary Science Advances). Each kind of excitation can have low or high motor energy, similar to vehicles on a street, with the notable equation E=1/2 mv2, which is a parabola. In any case, for twist and charge, the majority of m are unique, and, since charges repulse thus can’t possess a similar state as another charge, there is two times as wide a scope of energy for charge with respect to turn. The outcomes measure energy as a component of the attractive field, which is comparable to force or speed, v, showing these two energy parabolas, which should be visible in places as far as possible, up to multiple times the most noteworthy energy involved by electrons in the framework.
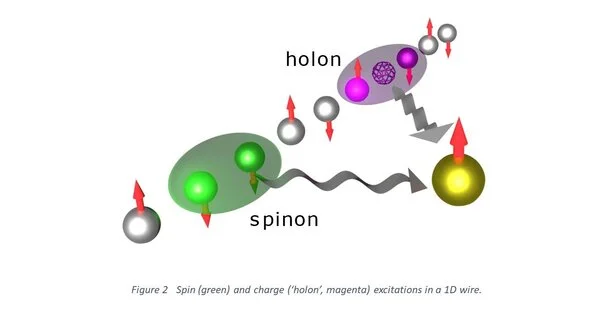
Figure 2. Turn (green) and charge (‘holon’, red) excitations in a 1D wire.
“Perhaps the vehicles (like charges) are moving slowly, but their travelers (like twists) are moving quickly,” explained Pedro Vianez, who did the calculations for his Ph.D. at the Cavendish Laboratory in Cambridge.”In any event, when the vehicles and travelers delay down or accelerate, they actually stay isolated!”
“What is surprising here is that we are done discussing electrons yet, all things being equal, about composite (quasi) particles of twist and charge — regularly named spinons and holons, individually. For quite a while, these were accepted to become shaky at such high energies, yet what is noticed focuses on the very inverse — they appear to act in a manner basically the same as expected; free, stable electrons, each with their own mass. Then again, actually they are not, as a matter of fact, electrons, however excitations of an entire ocean of charges or twists! ” Oleksandr Tsyplyatyev, the scholar who led the work at Goethe University in Frankfurt,
“This paper addresses the climax of nearly 10 years of trial and hypothetical work on the material science of one-layered frameworks,” said Chris Ford, who led the exploratory group. “We were generally inquisitive to see what might happen assuming we took the framework to higher energies, so we logically further developed our estimation goal to choose new elements. We created a progression of semiconducting varieties of wires going from 1 to 18 microns long (that is, down to a thousandth of a millimeter or roughly multiple times more slender than a human hair), with as many as 30 electrons in a wire, and estimated them at 0.3 K (or as such, -273.85 C, multiple times colder than space). “
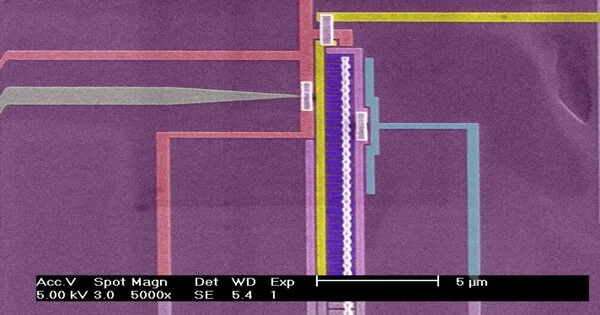
Figure 3a. Checking electron micrographs of a gadget, showing the different doors used to characterize the 1D wires (Part 1).
Details on experimental technique
Electrons burrow from the 1D wires into a contiguous two-layered electron gas, which goes about as a spectrometer, delivering a guide of the connection between energy and force. “This procedure is all basically the same as point settled photoemission spectroscopy (ARPES), which is an ordinarily involved technique for deciding the band design of materials in consolidated matter physical science.” That’s what the key distinction is. Instead of testing at the surface, our framework is covered 100 nanometers underneath it, “said Vianez. This permitted the specialists to accomplish their goal and control the extraordinary for this kind of spectroscopy analysis.
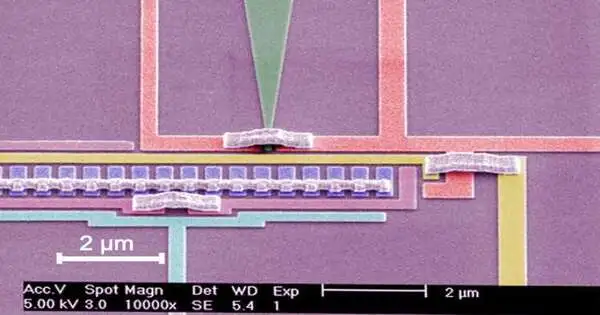
Figure 3b. Examining electron micrographs of a gadget, showing the different doors used to characterize the 1D wires (Part 2).
Conclusion
These outcomes presently open the question of whether or not this twist charge partition of the entire electron ocean stays vigorous past 1D, e.g., in high-temperature superconducting materials. It can now be used to rationale devices that outfit turn (spintronics), which offer a significant decrease (by three significant degrees!) in the energy utilization of a semiconductor while also working on how we might interpret quantum matter and providing another instrument for designing quantum materials.
More information: Pedro M. T. Vianez et al, Observing separate spin and charge Fermi seas in a strongly correlated one-dimensional conductor, Science Advances (2022). DOI: 10.1126/sciadv.abm2781