The most common method of hydrogen synthesis is sustainable water splitting. Electrolysis, high-temperature electrolysis, pure and hybrid thermochemical cycles, and photochemical/radiochemical processes are among the five sustainable water-splitting technologies mentioned. A group of researchers has identified a new, sustainable, and practical way for creating hydrogen from water.
A team of researchers at the RIKEN Center for Sustainable Resource Science (CSRS) in Japan lead by Ryuhei Nakamura found a novel sustainable and feasible method for creating hydrogen from water. Unlike present approaches, the novel process does not necessitate the use of rare metals that are either expensive or scarce. Instead, cobalt and manganese, two relatively abundant metals, can now be used to make hydrogen for fuel cells and agricultural fertilizers. The findings were published in Nature Catalysis.
Unlike traditional fossil fuels, which emit carbon dioxide when used, hydrogen is a clean fuel that emits only water as a byproduct. The energy grid can be rendered clean, renewable, and sustainable if hydrogen can be produced from water using renewable electricity. Furthermore, hydrogen is a major component in the production of ammonia, which is utilized in almost all synthetic fertilizers. However, instead of collecting hydrogen from water in a clean manner, ammonia plants currently rely on fossil fuels to produce the hydrogen they require.
So, why do we continue to rely on fossil fuels? One issue is that the technique of extracting hydrogen, known as electrolysis, is costly and not yet sustainable.
A highly active and stable catalyst made of plentiful metals is used to produce hydrogen. In the long run, we believe this is a significant step toward establishing a sustainable hydrogen economy. We anticipate that the cost of green hydrogen technology, like that of other renewable technologies such as solar cells and wind power, will fall in the near future as additional improvements are achieved.
Ailong Li
“This is mostly due to a scarcity of good catalysts,” Nakamura explains. “The catalyst must be exceedingly active in addition to being able to resist the strong acidic environment. If this is not done, the quantity of electricity required for the reaction to produce a given amount of hydrogen skyrockets, and so does the cost.”
The most active catalysts for water electrolysis are currently rare metals such as platinum and iridium, which causes a quandary because they are pricey and classified as “endangered species” among metals. Switching the entire globe to hydrogen fuel right now would require approximately 800 years of iridium manufacturing, an amount that may or may not exist. Conversely, plentiful metals such as iron and nickel are insufficiently active and tend to dissolve promptly in the severe acidic electrolysis environment.
The researchers investigated combined cobalt and manganese oxides in their search for a superior catalyst. Cobalt oxides can be active in the necessary process, although they corrode quickly in an acidic environment. Manganese oxides are more stable, but not nearly as active. The researchers thought that by mixing them, they would be able to take advantage of their complementing features. They also had to take into account the high current density required for actual application outside of the laboratory.
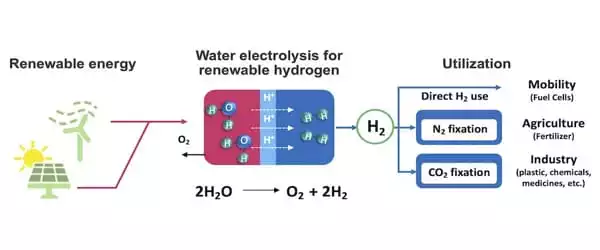
“We needed to set our study’s goal current density to around 10 to 100 times higher than what had been employed in previous studies for industrial scale hydrogen production,” adds co-first author Shuang Kong. “The large currents caused a number of issues, including physical disintegration of the catalyst.”
Eventually, via trial and error, the researchers developed an active and stable catalyst by introducing manganese into the spinel lattice of Co3O4, resulting in the mixed cobalt manganese oxide Co2MnO4.
Co2MnO4 fared admirably in testing. The activation levels were comparable to those of cutting-edge iridium oxides. Furthermore, the novel catalyst lasted more than two months at a current density of 200 milliamperes per square centimeter, indicating that it could be useful in practice. In comparison to other non-rare metal catalysts, which normally only last days or weeks at far lower current densities, the new electrocatalyst could be a game changer.
“We have accomplished what scientists have sought for decades,” adds co-first author Ailong Li. “A highly active and stable catalyst made of plentiful metals is used to produce hydrogen. In the long run, we believe this is a significant step toward establishing a sustainable hydrogen economy. We anticipate that the cost of green hydrogen technology, like that of other renewable technologies such as solar cells and wind power, will fall in the near future as additional improvements are achieved.”
The next stage in the lab will be to identify ways to extend the new catalyst’s lifetime and enhance its activity levels even further. “There is always room for improvement,” Nakamura adds, “and we will continue to search for a non-rare metal catalyst that can equal the performance of present iridium and platinum catalysts.”