Chemists have integrated computer functionality into rolling DNA-based motors, opening up a whole new world of possibilities for molecular robots in miniature. The first DNA-based motors that combine computational power with the capacity to burn fuel and move in an intentional direction were published in Nature Nanotechnology.”Beyond
getting the DNA motors to execute logic computations, one of our significant contributions is finding a mechanism to transform that information into a simple output signal—motion or no motion.” “Anyone with a cell phone outfitted with a cheap magnifying attachment may interpret this signal.””
Selma’s finding removes major hurdles that stood in the way of making DNA computers usable and feasible for a variety of biological applications, “says Khalid Salaita, senior author of the publication and Emory University professor of chemistry.” Salaita is also on the faculty of Georgia Tech’s and Emory’s Wallace H. Coulter Department of Biomedical Engineering.
“One of our big innovations, beyond getting the DNA motors to perform logic computations, is finding a way to convert that information into a simple output signal — motion or no motion,”
Selma Piranej, an Emory University PhD candidate in chemistry
The motors can detect chemical information in their surroundings, interpret it, and then respond appropriately, imitating certain basic qualities of biological cells.
“Previous DNA computers did not have built-in directed mobility,” Salaita says. However, in order to perform more complex tasks, you must mix computation and directed motion. Our DNA computers are essentially self-contained robots with sensing capabilities that determine whether or not they move.
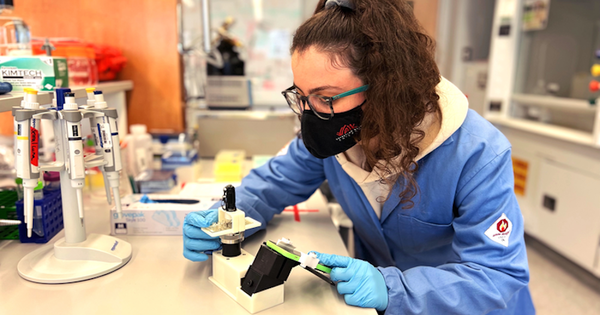
Because the motors can be designed to respond to a specific virus or DNA sequence, they have the potential to be used in medical testing and diagnostics.
Another significant advancement is that while deployed as a group, each motor can function independently and under separate programs. This paves the way for a single huge array of micron-sized motors to carry out a range of functions and communicate with one another.
Salaita explains that the capacity of the DNA motors to communicate with one another is a step toward achieving the kind of complicated, collective movement produced by swarms of ants or bacteria. “It may even result in emergent features.”
In DNA nanotechnology, the intrinsic inclination of the DNA bases A, G, C, and T to pair together is exploited. Scientists can manipulate the sequence of letters on synthetic DNA strands to cause the strands to join together in ways that form diverse structures and even functional machines.
In 2015, the Salaita lab, a biophysics and nanotechnology leader, created the first rolling DNA-based motor. The gadget was 1,000 times quicker than any other synthetic motor, propelling the science of molecular robotics forward. Because of its fast speed, it can be captured on video by a simple smart phone microscope.
The motor’s “chassis” is a micron-sized glass sphere. Hundreds of DNA strands, or “legs,” are permitted to bond to the sphere. These DNA legs are placed on a glass slide that has been coated with the reactant RNA, the motor’s fuel. The DNA legs are drawn to the RNA, but as soon as they touch it, they erase it due to the action of an enzyme that is attached to the DNA and kills only the RNA. As the legs bind and then release from the substrate, they continue to propel the sphere forward.
When Piranej joined the Salaita lab in 2018, she began working on a project to take rolling motors to the next level by incorporating computer programming logic.
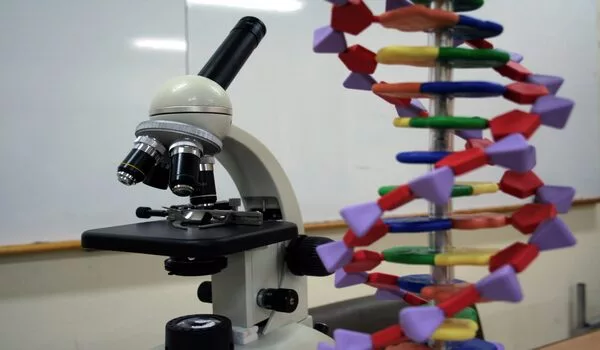
“Using DNA for computation is a big priority in the biomedical field,” Piranej explains. “I love the idea of engineering new types of technology using something that is innate in all of us.”
DNA functions as a biological computer chip, storing massive amounts of data. Short strands of synthetic DNA serve as the basic units of operation for DNA computation. Researchers can alter the “software” of DNA by modifying the AGTC sequences on the strands.”DNA-based
computers and motors, unlike hard silicon chips, can function in water and other liquid conditions.” One of the major issues in the fabrication of silicon computer chips is trying to compress more data into a smaller and smaller footprint. DNA has the ability to conduct multiple processing activities in parallel in a very small space. The number of operations you may run at any given time could be infinite. “
Synthetic DNA is also biocompatible and inexpensive to produce. “You may copy and paste DNA as many times as you like using enzymes,” Salaita explains. It’s almost free.
However, limitations exist in the new field of DNA computation. Making the output of computations understandable is a significant challenge. Current methods rely mainly on labeling DNA with fluorescent compounds and then measuring the intensity of light emitted at various wavelengths. This procedure necessitates the use of costly and bulky machinery. It also restricts the kinds of signals that can be read to those found in the electromagnetic spectrum.
Piranej, who was trained as a chemist, began learning the fundamentals of computer science and delving into bioengineering literature in order to overcome this obstacle. She came up with the concept of performing the computation by utilizing a well-known reaction in bioengineering and coupling it with the motion of the rolling motors.
The event, known as toehold-mediated strand displacement, takes place on duplex DNA, which consists of two complementary strands. Except for one slack, floppy end of a strand known as the toe hold, the strands are closely clutching one another. By coating the rolling motor with duplex DNA that is complementary to a DNA target—a sequence of interest—the rolling motor can be programmed.
When the molecular motor comes into contact with the DNA target while rolling along its RNA track, the DNA target attaches to the duplex DNA’s toe hold, pulls it apart, and anchors the motor in place. The computer readout is reduced to “motion” or “no motion.”
“I made this extremely loud, delighted sound when I initially saw this concept function during an experiment,” Piranej recalls. “‘Are you all right?’ inquired one of my coworkers. Nothing beats watching your idea come to life in that way. That’s a fantastic opportunity.
These two basic logic gates of “motion” or “no motion” can be strung together to create more complex processes, similar to how normal computer programs are built on logic gates of “zero” or “one.”
Piranej took the research a step further by figuring out how to combine multiple computer activities while still being able to view the result. She merely changed the size and composition of the small spheres that serve as the chassis for the DNA-based rolling motors. For example, the spheres can be three to five microns in diameter and constructed of silica or polystyrene. Each modification results in slightly different optical properties, which may be recognized using a cell phone microscope.
The Salaita lab is attempting to form a partnership with scientists at the Atlanta Institution for Microsystems Engineered Point-of-Care Technologies, an NIH-funded center founded by Emory and Georgia Tech. They are investigating the feasibility of using DNA-computing technologies for home diagnostics of COVID-19 and other illness biomarkers.
“Developing gadgets for biomedical applications is very fulfilling since it gives me the opportunity to make a significant difference in people’s lives,” adds Piranej. “The project’s hurdles have made it more enjoyable for me,” she adds.
Emory University donated the materials for the story. Carol Clark wrote the original.