The FERMI satellite of NASA searches for extremely long-wavelength gravitational waves.
Low-frequency gravity waves are emitted by coalescing supermassive black holes in the centers of merging galaxies. Astronomers have been looking for these waves with big radio telescopes, looking for the tiny effect of these spacetime ripples on radio waves released by pulsars in our galaxy. Now, an international team of scientists has demonstrated that the Fermi Gamma-ray Space Telescope’s high-energy photons may also be employed in the hunt. Detecting gravitational waves with gamma rays rather than radio waves provides a clearer view of the pulsars and is an independent and complementary method. Aditya Parthasarathy and Michael Kramer of the Max Planck Institute of Radio Astronomy in Bonn, Germany, led an international team of scientists who just published their findings in the journal Science.
“Fermi studies the universe in gamma rays, the most energetic form of light. We’ve been surprised at how good it is at finding the types of pulsars we need to look for these gravitational waves—over 100 so far!”
Matthew Kerr, a research physicist at the U.S. Naval Research Laboratory in Washington
Gravitational Waves in the Sea
A supermassive black hole lurks at the center of most galaxies, which are made up of hundreds of billions of stars like our own Milky Way. Galaxies are attracted to one another by their massive gravitational fields, and when they merge, their black holes sink to the new center. Long gravitational waves are created as black holes spiral inward and combine, stretching hundreds of trillions of kilometers between wave crests. Such merging supermassive black holes abound in the universe, causing a sea of low-frequency spacetime ripples.
Observing the pulses from pulsars, the dense relics of huge stars, astronomers have been looking for these waves for decades. Pulsars rotate with a high degree of regularity, and astronomers can predict when each pulse will occur. On the other hand, the sea of gravitational waves changes slightly when the pulses reach the planet, and this can be detected by carefully monitoring several pulsars across the sky.
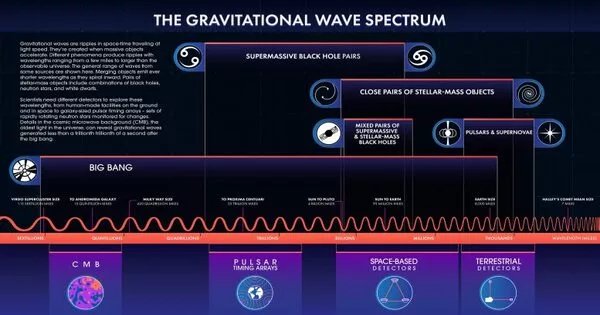
As two black holes (black spheres) of roughly similar mass spiral together and combine, gravitational waves are released by them. The strong curvature of space-time in the region is illustrated by the yellow structures surrounding the black holes. The orange ripples are space-time distortions generated by the fast circling masses. These distortions propagate and lessen, eventually transforming into gravitational waves (purple).
The time it takes for two black holes to combine is determined by their masses. The orbital period at the start of the movie for a system comprising black holes with about 30 times the mass of the sun, similar to the one identified by LIGO in 2015, is barely 65 milliseconds, with the black holes travelling at about 15% of the speed of light. Space-time distortions radiate orbital energy away, causing the pair to rapidly compress. As the two black holes get closer, they merge into a single black hole, which enters its “ringdown” phase, which is when the final gravitational waves are emitted. These events occurred in less than a quarter of a second during the 2015 LIGO detection. The Pleiades supercomputer at NASA’s Ames Research Center was used to run this simulation. NASA/Bernard J. Kelly (Goddard and University of Maryland Baltimore County), Chris Henze (Ames), and Tim Sandstrom (Goddard and University of Maryland Baltimore County) (CSC Government Solutions LLC)
Previously, enormous radio telescopes were employed to collect and analyze radio waves in order to find these waves. However, an international team of scientists has examined more than ten years of data collected by NASA’s Fermi Gamma-ray Space Telescope in search of these minute fluctuations, and their findings suggest that identifying these waves may be doable with just a few years of additional monitoring.
Gamma rays, the most energetic kind of light, are used by Fermi to study the universe. We’ve been shocked by how good it is at finding the types of pulsars we need to check for gravitational waves—over 100 so far! “, said Matthew Kerr, a research physicist at the United States Naval Research Laboratory in Washington. “Fermi and gamma rays have several unique properties that, when combined, make them an extremely strong instrument in our inquiry.”
The study’s findings were reported in the April 07 issue of Science by Kerr and Aditya Parthasarathy, a researcher at the Max Planck Institute for Radio Astronomy (MPIfR) in Bonn, Germany.
Cosmic Timepieces
Light manifests itself in a variety of ways. Low-frequency radio waves can pass through some materials, whereas high-frequency gamma rays collide with matter and explode into intense particle showers. Gravitational waves have a broad spectrum as well, with larger objects producing longer waves.
Because it is hard to build a detector large enough to detect the trillion-kilometer waves produced by merging supermassive black holes, astronomers rely on naturally existing detectors known as pulsar timing arrays. These are groups of millisecond pulsars that shine in both radio waves and gamma rays and rotate hundreds of times per second. These radiation beams appear to pulse regularly as they sweep across the world, and as they pass through the sea of gravitational waves, they are imprinted with the faint rumbling of faraway, gigantic black holes.
A Spectacular Probe
Pulsars were found using radio telescopes, and radio telescope pulsar timing array experiments have been running for nearly two decades. These large dishes are the most sensitive to gravity wave effects, although interstellar effects hamper radio data interpretation. Although space is mainly empty, radio waves contact many electrons as they travel the huge distance between a pulsar and the Earth. Interstellar electrons bend radio waves and change their arrival time in the same manner as a prism bends visible light. Because energetic gamma rays are not influenced in this way, they can be used to time pulsars in a complementary and independent manner.
Parthasarathy added, “When it comes to potentially detecting the gravitational wave background, the Fermi results are already 30% as excellent as the radio pulsar timing arrays.” “After another five years of pulsar data collecting and processing, it’ll be just as capable, with the extra benefit of not having to worry about all those stray electrons.”
A gamma-ray pulsar timing array, which was not anticipated before Fermi’s launch, represents a significant new capacity in gravitational wave astronomy.
“Detecting the gravitational wave background with pulsars is doable but tricky.” “An independent method, demonstrated here unexpectedly through Fermi, is fantastic news, both for confirming future findings and demonstrating its synergies with radio experiments,” says Michael Kramer, director of the MPIfR and head of its Fundamental Physics in Radio Astronomy research department.
The Fermi-LAT Collaboration published “A gamma-ray pulsar timing array constrains the nanohertz gravitational wave background” in Science on April 7, 2022.