Gigatons of ozone-harming substances are caught under the ocean bottom, and that is something worth being thankful for. Around the shorelines of the landmasses, where inclines sink down into the ocean, minuscule enclosures of ice trap methane gas, keeping it from getting away and rising into the climate.
While seldom in the news, these ice confinement arrangements, known as methane clathrates, definitely stand out on account of their capability to influence environmental change. During seaward penetrating, methane ice can stall out in pipes, making them freeze and burst. The 2010 Deepwater Skyline oil slick is remembered to have been brought about by the development of methane clathrates.
Yet, as of not long ago, the organic interaction behind how methane gas stays stable under the ocean has been totally obscure. In a leading-edge review, a cross-disciplinary group of Georgia Tech scientists found a formerly obscure class of bacterial proteins that assume a critical role in the development and soundness of methane clathrates.
“We were extremely fortunate that this worked, because despite the fact that we chose these proteins based on their similarity to antifreeze proteins, they are completely different. They perform a comparable role in nature, but through an entirely different biological mechanism, which I believe stimulates individuals.”
Abigail Johnson, a former Ph.D. student in Glass’ lab and co-first author on the paper,
A group led by Jennifer Glass, academic administrator in the School of Earth and Barometrical Sciences, and Raquel Lieberman, teacher and Sepcic-Pfeil Seat in the School of Science and Organic Chemistry, showed that these original bacterial proteins stifle the development of methane clathrates as well as business synthetic compounds right now utilized in penetrating, yet are non-poisonous, eco-accommodating, and versatile. Their review illuminates the quest for life in the planetary group and could likewise build the wellbeing of moving flammable gas.
The examination, distributed in the diary PNAS Nexus, highlights the significance of essential science in concentrating on Earth’s normal organic frameworks and features the advantages of joint effort across disciplines.
“We needed to comprehend how these arrangements were remaining stable under the ocean bottom and how unequivocally the thing components were adding to their dependability,” Glass said. “This is the kind of thing nobody has done previously.”
Filtering through silt
The work began with the group inspecting an example of mud-like dregs that Glass obtained from the ocean bottom off the shore of Oregon.
Glass conjectured that the dregs would contain proteins that impact the development of methane clathrate and that those proteins would look like notable radiator fluid proteins in fish, which assist them with making due in cool conditions.
Yet, to affirm her speculation, Glass and her exploration group would initially need to recognize protein up-and-comers out of millions of potential targets contained in the dregs. They would then have to make the proteins in the lab; however, there was no comprehension of how these proteins could act. Additionally, nobody had worked with these proteins previously.
Glass moved toward Lieberman, whose lab concentrates on the construction of proteins. The initial step was to utilize DNA sequencing matched with bioinformatics to distinguish the qualities of the proteins contained in the silt. Dustin Huard, a specialist in Lieberman’s lab and the first creator of the paper, then pre-arranged competitor proteins that might actually tie to the methane clathrates. Huard utilized X-beam crystallography to determine the design of the proteins.
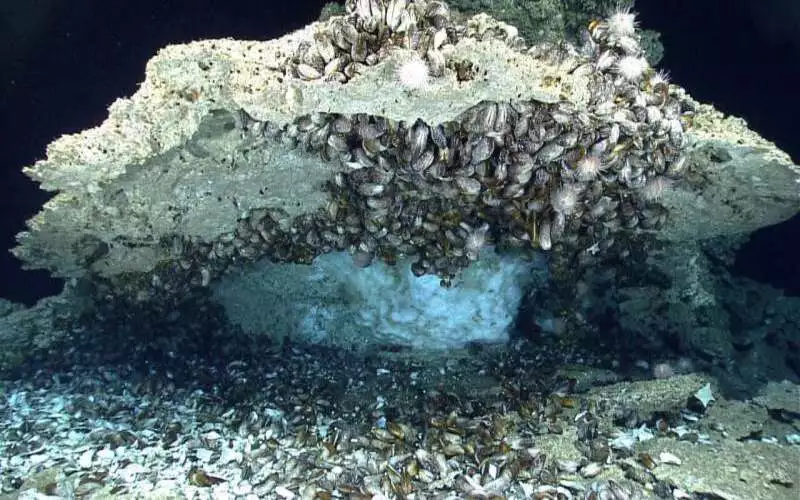
Methane clathrate (a white, ice-like material) was found under a stone from the ocean bottom of the northern Inlet of Mexico. Stores, for example, show that methane and different gases cross the ocean bottom and enter the sea. Photo credit: NOAA.
Making ocean bottom conditions in the lab
Huard gave the protein possibility to Abigail Johnson, a previous Ph.D. understudy in Glass’ lab and co-first creator on the paper, who is presently a postdoctoral specialist at the College of Georgia. To test the proteins, Johnson framed methane clathrates herself by reproducing the high tension and low temperature of the ocean bottom in the lab. Johnson worked with Sheng Dai, an academic administrator in the School of Common and Natural Designing, to construct a remarkable strain chamber without any preparation.
Johnson set the proteins in the tension vessel and changed the framework to impersonate the strain and temperature conditions expected for clathrate development. By compressing the vessel with methane, Johnson constrained methane into the drop, which made a methane clathrate construction structure.
She then, at that point, estimated how much gas was consumed by the clathrate—a mark of how rapidly and how much the clathrate shaped—and did so within sight of the proteins versus no proteins. Johnson found that with the clathrate-restricting proteins, less gas was consumed, and the clathrates softened at higher temperatures.
When the group approved that the proteins influence the arrangement and strength of methane clathrates, they utilized Huard’s protein gem design to complete sub-atomic element reenactments with the assistance of James (JC) Gumbart, a teacher in the School of Material Science. The reproductions permitted the group to recognize the particular site where the protein ties to the methane clathrate.
A shockingly original framework
The review revealed surprising insights into the construction and capability of the proteins. The specialists at first thought the piece of protein that was like fish radiator fluid proteins would play a part in clathrate-restricting. Shockingly, that piece of the protein didn’t assume a part, and something else entirely coordinated the communications.
They found that the proteins don’t tie to ice but instead cooperate with the clathrate design itself, coordinating its development. In particular, the piece of the protein that had comparative qualities to liquid catalyst proteins was covered in the protein structure and, on second thought, assumed a part in settling the protein.
The analysts found that the proteins performed better at adjusting methane clathrate than any of the radiator fluid proteins that had been tried previously. They performed similarly as well as, while perhaps worse than, the harmful business clathrate inhibitors as of now utilized in boring that present serious natural dangers.
Forestalling clathrate development in flammable gas pipelines is a billion-dollar industry. On the off chance that these biodegradable proteins could be utilized to forestall flammable gas spills, it would incredibly decrease the risk of natural harm.
“We were fortunate to such an extent that this really worked, on the grounds that despite the fact that we picked these proteins in light of their comparability to radiator fluid proteins, they are totally unique,” Johnson said. “They have a comparative capability in nature; however, they do so through something else entirely, and I believe that truly energizes individuals.”
Methane clathrates likely exist all through the nearby planet group—on the subsurface of Mars, for instance, and on cold moons in the external planetary group, like Europa. That’s what the group’s discoveries demonstrate: assuming microorganisms exist on other planetary bodies, they could deliver comparable biomolecules to hold fluid water in diverts in the clathrate that could support life.
“We’re actually finding out such a huge amount about the essential frameworks on our planet,” Huard said. “That is an incredible aspect concerning Georgia Tech—various networks can meet up to do truly cool, unforeseen science. I never figured I would be chipping away at an astrobiology project; however, we are right here, and we’ve been extremely effective.”
More information: Dustin J E Huard et al, Molecular basis for inhibition of methane clathrate growth by a deep subsurface bacterial protein, PNAS Nexus (2023). DOI: 10.1093/pnasnexus/pgad268