Stanford University specialists have fostered a critical trial gadget for future quantum material science-based advances that gets a page from current, regular mechanical gadgets.
Dependable, minimized, sturdy, and proficient, acoustic gadgets outfit mechanical movement to perform valuable errands. A perfect representation of such a gadget is the mechanical oscillator. Whenever uprooted by a power source—like sound, for example—parts of the gadget start moving to and for about their unique position. Making this intermittent movement is a convenient method for keeping time, channeling signals, and sensing movement in pervasive gadgets, including telephones, PCs, and watches.
Specialists have tried to bring the advantages of mechanical frameworks down into the minuscule size of the secretive quantum domain, where iotas carefully cooperate and act in unreasonable ways. Toward this end, Stanford specialists led by Amir Safavi-Naeini have exhibited new abilities by coupling little nanomechanical oscillators with a kind of circuit that can store and handle energy as a qubit, or quantum “bit” of data. Utilizing the gadget’s qubit, the scientists can control the quantum condition of mechanical oscillators, creating the sorts of quantum mechanical impacts that could sometimes enable progress in registering and ultraprecise detection frameworks.
“With this device, we’ve shown an important next step in trying to build quantum computers and other useful quantum devices based on mechanical systems,”
Safavi-Naeini, an associate professor in the Department of Applied Physics at Stanford’s School of Humanities and Sciences.
With this gadget, “we’ve shown a significant next step in attempting to construct quantum PCs and other valuable quantum gadgets in view of mechanical frameworks,” said Safavi-Naeini, an academic partner in the Department of Applied Physics at Stanford’s School of Humanities and Sciences. Safavi-Naeini is the senior creator of another review distributed on April 20 in the diary Nature, portraying the discoveries. “We’re fundamentally hoping to assemble’mechanical quantum mechanical’ frameworks,” he said.
Marshaling quantum impacts on CPUs
The joint first creators of the review, Alex Wollack and Agnetta Cleland, both Ph.D. competitors at Stanford, led the work to foster this new mechanics-based quantum equipment. Utilizing the Stanford Nano Shared Facilities nearby, the scientists worked in cleanrooms while furnished in the body-covering white “rabbit suits” worn at semiconductor fabricating plants to keep debasements from tainting the delicate materials in play.
With particular gear, Wollack and Cleland manufactured equipment parts at nanometer-scale goals onto two silicon microprocessors. The specialists then, at that point, stuck the two chips together so the parts on the base chip confronted those on the top half, sandwich-style.
On the base chip, Wollack and Cleland designed an aluminum superconducting circuit that frames the gadget’s qubit. Sending microwave beats into this circuit produces photons (particles of light), which encode a qubit of data in the gadget. Unlike conventional electrical devices, which store bits as voltages addressing either a 0 or a 1, qubits in quantum mechanical devices can address weighted combinations of 0 and 1 at the same time.This is a result of the quantum mechanical peculiarity known as superposition, where a quantum framework exists in various quantum states without a moment’s delay until the framework is estimated.
“The manner in which reality works at the quantum mechanical level is altogether different from our naturally visible experience of the world,” said Safavi-Naeini.
The top chip contains two nanomechanical resonators framed by suspended, precious stone designs only two or three nanometers long, or billionths of a meter long. The gems are made of lithium niobate, a piezoelectric material. Materials with this property can change electrical power into movement, which, on account of this gadget, implies the electric field conveyed by the qubit photon is changed into a quantum (or a solitary unit) of vibrational energy called a phonon.
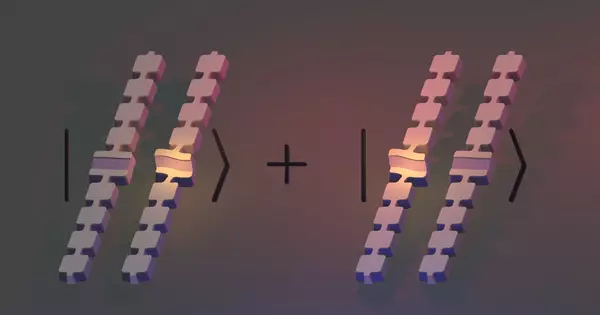
“Very much like light waves, which are quantized into photons, sound waves are quantized into ‘particles’ called phonons,” said Cleland, “and by joining the energy of these various structures in our gadget, we make a cross-breed quantum innovation that bridles the upsides of both.”
The age of these phonons permitted each nanomechanical oscillator to behave like a register, which is the tiniest potential information-holding component in a PC, and with the qubit providing the information. Like the qubit, the oscillators can also be in a superposition state—they can be both energized (addressing 1) and not invigorated (addressing 0) simultaneously. The superconducting circuit empowered the scientists to plan, read out, and adjust the information put away in the registers, thoughtfully like how traditional (non-quantum) PCs work.
“The fantasy is to make a gadget that works similarly to silicon CPUs, for instance, in your telephone or on a thumb drive, where registers store bits,” said Safavi-Naeini. And keeping in mind that we can’t store quantum bits on a thumb drive right now, we’re showing a similar kind of thing with mechanical resonators.”
Utilizing entrapment
In past superposition, the association between the photons and resonators in the gadget further utilized another significant quantum mechanical peculiarity called entrapment. What makes ensnared states so strange, and furthermore, notoriously challenging to make in the lab, is that the data about the condition of the framework is appropriated across various parts. In these frameworks, it is feasible to have a deep understanding of two particles together, but nothing around one of the particles is noticed separately. Envision two coins that are flipped in two better places and that are seen to land as heads or tails arbitrarily with equivalent likelihood. When estimations at the better places are looked at, they generally correspond; that is, assuming one coin lands as tails, the other coin is guaranteed to land as heads.
The control of different qubits, all in superposition and snared, is the one-two punch controlling calculation and detecting sought-after quantum-based advancements. Without superposition and bunches of traps, you can’t construct a quantum PC,” said Safavi-Naeini.
To exhibit these quantum impacts in the trial, the Stanford analysts produced a solitary qubit, put away as a photon in the circuit on the base chip. The circuit was then permitted to trade energy with one of the mechanical oscillators on the top chip prior to moving the leftover data to the second mechanical gadget. By trading energy along these lines—first with one mechanical oscillator, and afterward with the subsequent oscillator—the specialists involved in the circuit used the circuit as an instrument to quantum precisely catch the two mechanical resonators with one another.
“The strangeness of quantum mechanics is on full display here,” said Wollack. “Not at all, sounds are discrete units, yet a single molecule of sound can be divided among the two entrapped plainly visible articles, each with trillions of iotas moving — or not moving — in show.”
Because, after finally performing pragmatic estimations, the time of supported ensnarement, or soundness, would be essentially longer—on the request for seconds rather than the small parts of seconds accomplished up to this point.Superposition and entrapment are both extremely vulnerable conditions, vulnerable to even minor aggravations like heat or other energy, and they also endow proposed quantum-detecting gadgets with dazzling responsiveness.However, Safavi-Naeini and his co-writers accept that longer cognizance times can be promptly reached by sharpening the manufacturing processes and advancing the materials in question.
“We’ve worked on the presentation of our framework throughout recent years, almost multiple times consistently,” said Safavi-Naeini. “Pushing ahead, we will keep on making substantial strides toward conceiving quantum mechanical gadgets, similar to PCs and sensors, and bring the advantages of mechanical frameworks into the quantum area.”