Quantum technology and research are creating a slew of new opportunities and possibilities. One of these possibilities involves communication speed, prompting experts to consider whether quantum communication is faster than the speed of light.
The key to maximizing traditional or quantum computing speeds lies in our ability to understand how electrons behave in solids, and a collaboration between the University of Michigan and the University of Regensburg captured electron movement in attoseconds – the fastest speed yet.
Seeing electrons move in one quintillionth of a second increments could help push processing speeds up to a billion times faster than what is currently possible. Furthermore, the research provides a “game-changing” tool for the study of many-body physics.
“Your current computer’s processor operates in gigahertz, that’s one billionth of a second per operation,” said Mackillo Kira, U-M professor of electrical engineering and computer science, who led the theoretical aspects of the study published in Nature. “In quantum computing, that’s extremely slow because electrons within a computer chip collide trillions of times a second and each collision terminates the quantum computing cycle.
“What we’ve needed, in order to push performance forward, are snapshots of that electron movement that are a billion times faster. And now we have it.”
Our solid-state attoclock has the potential to be a game changer, allowing us to design novel quantum materials with more precisely tailored properties and contribute to the development of new materials platforms for future quantum information technology.
Mackillo Kira
Rupert Huber, professor of physics at the University of Regensburg and corresponding author of the study, said the result’s potential impact in the field of many-body physics could surpass its computing impact.
“Many-body interactions are the microscopic driving forces behind the most coveted properties of solids, ranging from optical and electronic feats to intriguing phase transitions,” explained Huber, who led the experiment. “Our solid-state attoclock has the potential to be a game changer, allowing us to design novel quantum materials with more precisely tailored properties and contribute to the development of new materials platforms for future quantum information technology.”
Researchers typically use short bursts of focused extreme ultraviolet (XUV) light to observe electron movement within two-dimensional quantum materials. These bursts can reveal the activity of electrons attached to the nucleus of an atom. However, the large amounts of energy carried in those bursts prevent clear observation of electrons traveling through semiconductors, such as those used in current computers and materials under development for quantum computers.
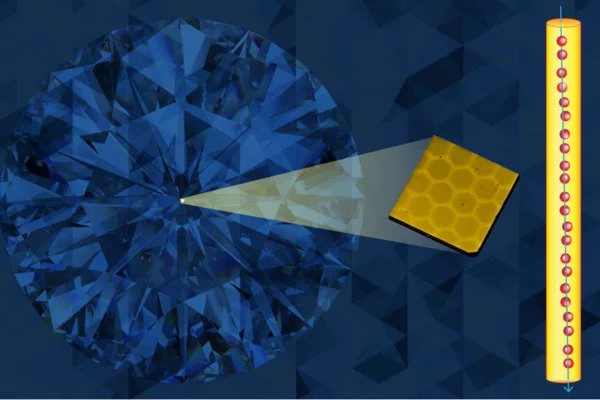
U-M engineers and collaborators use two light pulses with energy scales that correspond to those movable semiconductor electrons. The first, an infrared light pulse, places electrons in a state that allows them to move through the material. The following pulse, a lower-energy terahertz pulse, forces those electrons into controlled head-on collision trajectories. The collisions generate light bursts, the precise timing of which reveals interactions underlying quantum information and exotic quantum materials alike.
“We used two pulses: one that is energetically matched with the electron’s state and another that causes the state to change,” Kira explained. “We can basically film how these two pulses change the quantum state of the electron and then express that as a function of time.”
The two-pulse sequence allows time measurement with a precision better than one percent of the oscillation period of the terahertz radiation that accelerates the electrons.
“This is really unique and took us many years of development,” Huber said. “It is quite unexpected that such high-precision measurements are even possible if you remember how ridiculously short a single oscillation cycle of light is — and our time resolution is one hundred times faster yet.”
Quantum materials could have strong magnetic, superconductive, or superfluid phases, and quantum computing has the potential to solve problems that would take too long to solve on traditional computers. By pushing such quantum capabilities, we will eventually be able to solve problems that are currently out of our reach. This begins with fundamental observational science.
“No one has been able to build a scalable and fault-tolerant quantum computer so far, and we don’t even know what that would look like,” said Markus Borsch, a doctoral student in electrical and computer engineering at the University of Michigan. “However, basic research, such as studying how electronic motion in solids works at the most fundamental levels, may provide us with an idea that points us in the right direction.”