A multi-institutional team has developed an effective method for measuring high-dimensional qudits encoded in quantum frequency combs, a type of photon source, on a single optical chip using existing experimental and computational resources.
Although the word “qudit” may appear to be a misspelling, this lesser-known cousin of the qubit, or quantum bit, can carry more information and is more resistant to noise – both of which are important qualities required to improve the performance of quantum networks, quantum key distribution systems, and, eventually, the quantum internet.
Because of superposition, which is a phenomenon that allows multiple quantum states to exist at the same time, qubits can hold values of one, zero, or both – simultaneously. The “d” in qudit represents the number of different levels or values that a photon can encode. Qubits have two levels by default, but adding more levels transforms them into qudits.
Researchers from the US Department of Energy’s Oak Ridge National Laboratory, Purdue University, and the Swiss Federal Institute of Technology Lausanne, or EPFL, recently completed the full characterization of an entangled pair of eight-level qudits that formed a 64-dimensional quantum space, quadrupling the previous record for discrete frequency modes. These findings were published in the journal Nature Communications.
We combined state-of-the-art frequency-bin production with state-of-the-art light sources, and then used our technique to characterize high-dimensional qudit entanglement with a level of precision that hasn’t been shown before.
Joseph Lukens
“We’ve always known that it’s possible to encode 10- or 20-level qudits or even higher using the colors of photons, or optical frequencies, but the problem is that measuring these particles is very difficult,” said Hsuan-Hao Lu, a postdoctoral research associate at ORNL. “That’s the value of this paper — we found an efficient and novel technique that is relatively easy to do on the experimental side.”
Qudits are even more difficult to measure when they are entangled, meaning they share nonclassical correlations regardless of the physical distance between them. Despite these challenges, frequency-bin pairs two qudits in the form of photons that are entangled in their frequencies are well suited to carrying quantum information because they can follow a prescribed path through optical fiber without being significantly modified by their environment.
“We combined state-of-the-art frequency-bin production with state-of-the-art light sources, and then used our technique to characterize high-dimensional qudit entanglement with a level of precision that hasn’t been shown before,” said Joseph Lukens, a Wigner Fellow and research scientist at ORNL.
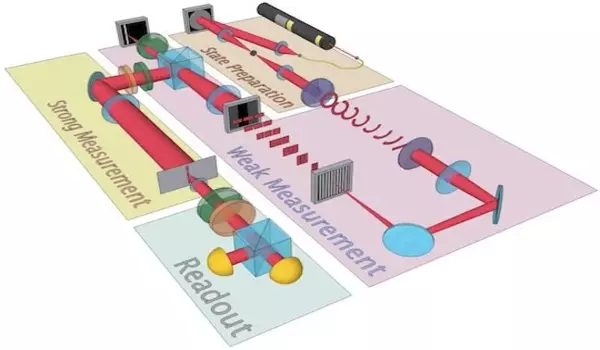
The researchers began their experiments by shining a laser into a micro-ring resonator, which is a circular, on-chip device designed by EPFL to generate nonclassical light. This powerful photon source occupies 1 square millimeter of space – roughly the size of a sharpened pencil point – and enabled the team to generate frequency-bin pairs in the form of quantum frequency combs.
Typically, qudit experiments necessitate the construction of a type of quantum circuit known as a quantum gate. In this case, however, the team used an electro-optic phase modulator to mix different light frequencies and a pulse shaper to change the phase of these frequencies. These techniques are studied extensively at the Ultrafast Optics and Optical Fiber Communications Laboratory led by Andrew Weiner at Purdue, where Lu studied before joining ORNL.
These optical devices are commonplace in the telecommunications industry, and the researchers performed these operations at random to capture many different frequency correlations. According to Lu, this process is like rolling a pair of six-sided dice and recording how many times each combination of numbers appears — but now the dice are entangled with each other.
“This technique, which involves phase modulators and pulse shapers, is heavily pursued in the classical context for ultrafast and broadband photonic signal processing and has been extended to the quantum avenue of frequency qudits,” Weiner said.
To work backward and infer which quantum states produced frequency correlations ideal for qudit applications, the researchers developed a data analysis tool based on a statistical method called Bayesian inference and ran computer simulations at ORNL. This accomplishment builds on the team’s previous work focused on performing Bayesian analyses and reconstructing quantum states.
The scientists are currently fine-tuning their measurement method in preparation for a series of experiments. They hope to test quantum communication protocols such as teleportation, which is a method of transporting quantum information, and entanglement swapping, which is the process of entangling two previously unrelated particles, by sending signals through optical fiber.
Purdue graduate student Karthik Myilswamy plans to bring the micro-ring resonator to ORNL, where the team will test these capabilities on the laboratory’s quantum local area network. “We can now perform other application-oriented experiments now that we have a method to efficiently characterize entangled frequency qudits,” Myilswamy said.