Quantum simulators are extremely powerful tools for understanding and simulating complex quantum systems that are difficult to study directly. They have the potential to transform fields like materials science, chemistry, and even optimization problems. If successfully scaled up, the team’s new system could help answer questions about superconductors and other unusual states of matter.
Some of the most fascinating topics in modern physics, such as high-temperature superconductors and some proposals for quantum computers, revolve around the strange things that occur when these systems oscillate between two quantum states.
Unfortunately, understanding what happens at those points, dubbed quantum critical points, has proven difficult. The math is frequently too difficult to solve, and today’s computers are not always up to the task of simulating what happens, particularly in systems involving a significant number of atoms.
We’re always making mathematical models that we hope will capture the essence of phenomena we’re interested in, but even if we believe they’re correct, they’re often not solvable in a reasonable amount of time.
David Goldhaber-Gordon
Researchers at Stanford University and the Department of Energy’s SLAC National Accelerator Laboratory, along with their colleagues, have taken a step toward developing a quantum simulator, a different approach. Although the new device only simulates the interactions of two quantum objects for the time being, the researchers argue in a paper published in Nature Physics that it could be easily scaled up. If so, researchers could use it to simulate more complicated systems and begin answering some of the most tantalizing questions in physics.
“We’re always making mathematical models that we hope will capture the essence of phenomena we’re interested in, but even if we believe they’re correct, they’re often not solvable in a reasonable amount of time” with current methods, said David Goldhaber-Gordon, a professor of physics at Stanford and a researcher with the Stanford Institute for Materials and Energy Sciences (SIMES). With a path toward a quantum simulator, he said, “we have these knobs to turn that no one’s ever had before.”
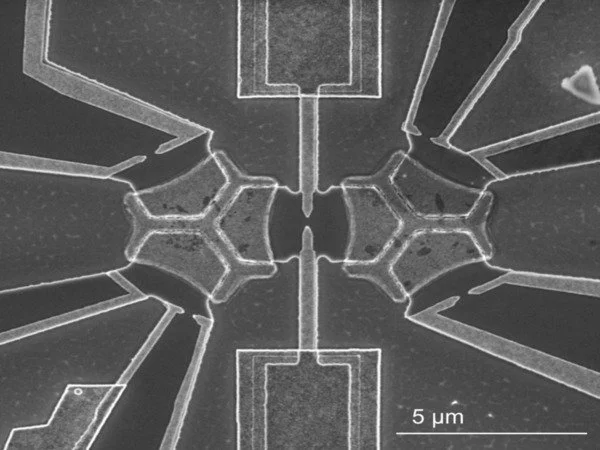
Researchers take a step toward novel quantum simulators
Islands in a sea of electrons
The basic concept of a quantum simulator, according to Goldhaber-Gordon, is similar to a mechanical model of the solar system, in which a crank is turned and interlocking gears rotate to represent the motion of the moon and planets. An “orrery” discovered in a shipwreck over 2000 years ago is thought to have produced quantitative predictions of eclipse timings and planetary locations in the sky, and analogous machines were used even into the late twentieth century for mathematical calculations that were too difficult for the most advanced digital computers at the time.
Researchers developing quantum simulators, like the designers of a mechanical model of a solar system, must ensure that their simulators align reasonably well with the mathematical models they are meant to simulate.
Many of the systems of interest to Goldhaber-Gordon and his colleagues, such as certain superconductors with quantum critical points, can be imagined as atoms of one element arranged in a periodic lattice embedded within a reservoir of mobile electrons. The lattice atoms in such a material are all identical, and they all interact with one another as well as with the sea of electrons that surrounds them.
To model materials like that with a quantum simulator, the simulator needs to have stand-ins for the lattice atoms that are nearly identical to each other, and these need to interact strongly with each other and with a surrounding reservoir of electrons. The system also needs to be tunable in some way, so that experimenters can vary different parameters of the experiment to gain insight into the simulation.
Most quantum simulation proposals, according to Winston Pouse, a graduate student in Goldhaber-Gordon’s lab and the paper’s first author, do not meet all of those requirements at once. “At a high level, there are ultracold atoms with identical atoms, but implementing a strong coupling to a reservoir is difficult.” Then there are quantum dot-based simulators, where we can achieve a strong coupling but not identical sites,” Pouse explained.
A possible solution, according to Goldhaber-Gordon, arose from the work of French physicist Frédéric Pierre, who was studying nanoscale devices in which an island of metal was situated between specially designed pools of electrons known as two-dimensional electron gases. The flow of electrons between the pools and the metal island was controlled by voltage-controlled gates.
A simple simulator
The team also realized that by connecting Pierre’s metal islands, they could create a simple system that should exhibit the quantum critical phenomenon they were looking for. Building the devices proved to be one of the most difficult aspects. First, the basic circuit outlines must be nanoscopically etched into semiconductors. Then, to create each metal island, someone must deposit and melt a tiny blob of metal onto the underlying structure.
“They’re very difficult to make,” said Pouse of the devices. “It’s not a super clean process, and it’s important to make good contact” between the metal and the underlying semiconductor.
Despite those difficulties, the team, whose work is part of broader quantum science efforts at Stanford and SLAC, was able to build a device with two metal islands and examine how electrons moved through it under a variety of conditions. Their results matched up with calculations which took weeks on a supercomputer — hinting that they may have found a way to investigate quantum critical phenomena much more efficiently than before.
“While we have not yet built an all-purpose programmable quantum computer with sufficient power to solve all of the open problems in physics,” said Andrew Mitchell, a theoretical physicist at University College Dublin’s Centre for Quantum Engineering, Science, and Technology (C-QuEST) and paper co-author, “we can now build bespoke analogue devices with quantum components that can solve specific quantum physics problems.”
Eventually, Goldhaber-Gordon hopes to build devices with more and more islands, allowing them to simulate larger and larger atomic lattices, capturing essential properties of real materials.
But first, they hope to improve the design of their two-island device. One goal is to reduce the size of the metal islands, which could allow them to operate at lower temperatures: cutting-edge ultralow temperature “refrigerators” can reach temperatures as low as a fiftyth of a degree above absolute zero, but that was barely cold enough for the experiment the researchers just completed. Another goal is to develop a more reliable method of creating islands than simply dripping molten metal onto a semiconductor.
However, once those kinks are ironed out, the researchers believe their work will lay the groundwork for significant advances in physicists’ understanding of certain types of superconductors, as well as possibly even more exotic physics, such as hypothetical quantum states that mimic particles with only a fraction of the charge of an electron.