Engineers can simplify the complex physics that describes fault movement by using single calcite crystals with varying surface roughness. Researchers from the University of Illinois Urbana-Champaign show how this simplification could lead to better earthquake prediction in a new study.
Scientists describe fault behavior using observational models that take into account the frictional coefficients of rocks and minerals. The fault strength is calculated using “rate-and-state” equations, which has implications for earthquake strength and frequency. However, because of the number of unique variables that must be considered for each fault, including the effect of water, applying these empirical models to earthquake prediction is impractical.
The study, led by civil and environmental engineering professor Rosa Espinosa- Marzal, looks at the relationship between friction and the surface roughness of calcite – one of the most common rock-forming minerals in Earth’s crust – to formulate a more theoretical approach to defining rate-and-state laws.
The findings are published in the Proceedings of the National Academy of Sciences.
Our findings are consistent with a recent study that found that water reduces fault strength when compared to dry conditions. Our findings are also consistent with another study that found that low-frequency earthquakes tend to occur along wet faults, implying that decreased friction caused by water may be a mechanism for slow earthquakes in some environments.
Espinosa-Marzal
“Our goal is to investigate the nanoscale processes that may cause fault movement,” said Binxin Fu, a CEE graduate student and the study’s first author. “The processes we study at the nanoscale are less complex than those studied at the macroscale. As a result, we intend to use microscopic observations to bridge the gap between the nanoscale and macroscale worlds in order to describe fault behavior with less complication.”
The roughness of a mineral crystal is primarily determined by its atomic structure. The rocks in contact zones, however, are scraped, dissolved, and annealed as they rub against each other, affecting their nanoscale texture, according to the researchers.
To test how nanoscale mineral roughness can affect fault behavior, the team prepared atomically smooth and rough calcite crystals in dry and wet environments to simulate dry rocks and those containing pore water. Atomic force microscopy measured friction by dragging a tiny, pressure-mounted silicon tip across different crystal surfaces exposed to simulated fault zone conditions: wet surface and smooth calcite; wet surface and rough calcite; dry surface and smooth calcite; and dry surface with rough calcite.
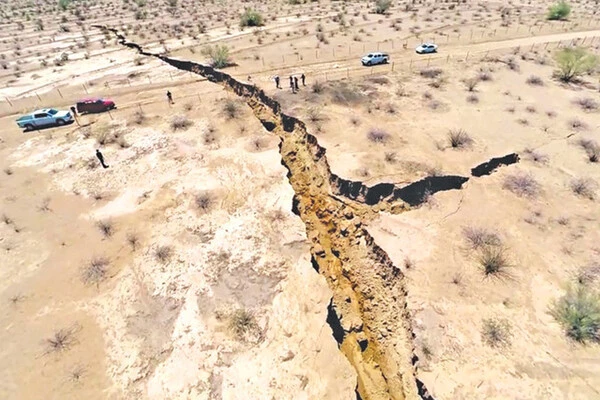
“Depending on the mineral types and the environment, friction can increase or decrease with sliding velocity,” Espinosa-Marzal explained. “We discovered that friction increases with sliding rate along rougher mineral surfaces in calcite, and even more in the presence of water. We reduce the analysis’s complexity and provide a fundamental understanding of the rate-and-state equations by using data from a common mineral type and a limited number of contact scenarios.”
The researchers compared their findings to studies from natural settings with calcite-containing rock at shallow crustal levels.
“Our findings are consistent with a recent study that found that water reduces fault strength when compared to dry conditions,” Espinosa-Marzal said. “Our findings are also consistent with another study that found that low-frequency earthquakes tend to occur along wet faults, implying that decreased friction caused by water may be a mechanism for slow earthquakes in some environments.”
This breakthrough could assist seismologists in redefining rate-and-state laws to determine where stress is building up in the crust – and provide clues as to where and when future earthquakes might occur.
The team acknowledges that many other factors, such as temperature and the influence of other common crustal minerals like quartz and mica, must still be considered. These variables will be included in future models, according to the researchers.