X-ray imaging techniques are used to study the structure and properties of materials on the atomic and molecular levels. One way to study the transient phases of quantum materials is to use time-resolved x-ray imaging, which involves taking a series of x-ray images at different times after the material has undergone some kind of perturbation. This can reveal information about the dynamics of the material’s electronic and structural properties and can help to shed light on the underlying quantum phenomena at play.
There are several different methods for performing time-resolved x-ray imaging, including pump-probe techniques, which involve using a laser or other light source to “pump” the material and then using x-rays to “probe” the response of the material at different times. Other methods include using x-ray scattering or diffraction techniques to study the dynamics of the material’s structure.
An international team of researchers recently demonstrated for the first time how to image phase transitions using a new lensless ultrafast X-Ray method. The direct observation of the dynamics of quantum materials at the nanoscale is now possible thanks to this new method.
The use of light to generate transient phases in quantum materials is quickly becoming a novel method of engineering new properties in them, such as superconductivity or nanoscale topological defects. However, due to the wide range of spatial and time scales involved in the process, visualizing the growth of a new phase in a solid is difficult.
Although in the last two decades, scientists have explained light-induced phase transitions by invoking nanoscale dynamics, real space images have not yet been produced and, thus, no one has seen them.
In the new study published in Nature Physics, ICFO researchers Allan S. Johnson and Daniel Pérez-Salinas, led by former ICFO Prof. Simon Wall, in collaboration with colleagues from Aarhus University, Sogang University, Vanderbilt University, the Max Born Institute, the Diamond Light Source, ALBA Synchrotron, Utrecht University, and the Pohang Accelerator Laboratory, have pioneered a new imaging method that allows the capture of the light-induced phase transition in vanadium oxide (VO2) with high spatial and temporal resolution.
Using our imaging method, we discovered that there was no link, at least in this case, between the picosecond dynamics we observed and any nanoscale changes or exotic phases. As a result, it appears that some of those conclusions will have to be reconsidered.
Allan Johnson
The researchers’ new technique is based on coherent X-ray hyperspectral imaging at a free electron laser, which has allowed them to visualize and better understand the insulator-to-metal phase transition in this well-known quantum material at the nanoscale.
The crystal VO2 has been widely used in the study of light-induced phase transitions. It was the first material to have its solid-solid transition tracked using time-resolved X-ray diffraction, and its electronic nature was studied for the first time using ultrafast X-ray absorption techniques. At room temperature, VO2 is in the insulating phase. However, by applying light to the material, it is possible to break the dimers of the vanadium ion pairs and drive the transition from an insulating to a metallic phase.
The authors of the study prepared thin samples of VO2 with a gold mask to define the field of view in their experiment. The samples were then taken to the Pohang Accelerator Laboratory’s X-ray Free Electron Laser facility, where an optical laser pulse induced the transient phase before being probed by an ultrafast X-ray laser pulse. The scattered X-rays were captured by a camera, and the coherent scattering patterns were converted into images using two methods: Fourier Transform Holography (FTH) and Coherent Diffractive Imaging (CDI) (CDI). Images were captured at various time delays and X-ray wavelengths to create a movie of the process with 150 femtosecond time resolution and 50 nm spatial resolution, as well as full hyperspectral data.
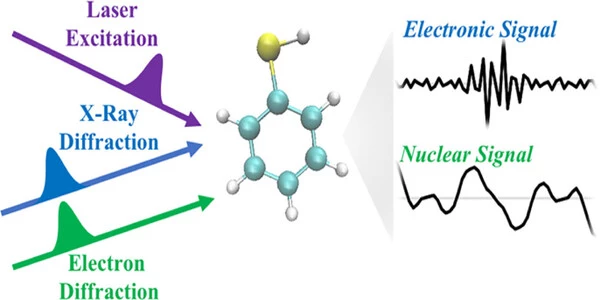
The surprising role of the pressure
The new methodology allowed the researchers to better understand the dynamics of the phase transition in VO2. They found that pressure plays a much larger role in light-induced phase transitions than previously expected or assumed.
“We discovered that the transient phases aren’t nearly as exotic as people thought! Instead of a truly non-equilibrium phase, we discovered that the ultrafast transition causes massive internal pressures in the sample that are millions of times higher than atmospheric. This pressure alters the material properties and takes time to relax, giving the impression of a transient phase” Allan Johnson, a postdoctoral researcher at ICFO, agrees. “Using our imaging method, we discovered that there was no link, at least in this case, between the picosecond dynamics we observed and any nanoscale changes or exotic phases. As a result, it appears that some of those conclusions will have to be reconsidered.”
The use of a hyperspectral image was critical in determining the role of pressure in the process. “By combining imaging and spectroscopy into one great image, we are able to retrieve much more information, allowing us to actually see detailed features and decipher where they come from,” Johnson continues. “This was critical in determining whether each part of our crystal was a normal or exotic out-of-equilibrium phase—and with this information, we were able to determine that during the phase transitions, all regions of our crystal were the same, except for the pressure.”
Challenging research
One of the most difficult challenges the researchers faced during the experiment was ensuring that the VO2 crystal sample returned to its original starting phase after each laser illumination. To ensure that this would happen, they conducted preliminary experiments at synchrotrons in which they took several crystal samples and repeatedly shone the laser on them to test their ability to recover to their original state.
The second challenge was having access to an X-Ray free electron laser, and large research facilities where the time windows to conduct the experiments are very competitive and in demand because there are only a few in the world. “We had to spend two weeks in quarantine in South Korea due to the COVID-19 restrictions before we got our one shot of just five days to make the experiment work, so that was an intense time” Johnson recalls.
Although the current work is described as fundamental research, the potential applications of this technique are diverse, as they could “look at polarons moving inside catalytic materials, try imaging superconductivity itself, or even help us understand novel nanotechnologies by viewing and imaging inside nanoscale devices,” Johnson concludes.