Utilizing a “creepy” peculiarity of quantum material science, Caltech specialists have found a method for multiplying the goal of a light magnifying lens.
A team led by Bren Professor of Medical Engineering and Electrical Engineering Lihong Wang demonstrates the achievement of a quantum entanglement-based leap forward in microscopy in a paper published in Nature Communications. A phenomenon known as quantum entanglement occurs when two particles are connected in such a way that the state of one particle is linked to the state of the other particle, regardless of how close the particles are to one another. Albert Einstein broadly alluded to the quantum trap as “creepy activity a ways off” in light of the fact that it couldn’t be made sense of by his relativity hypothesis.
Any kind of particle can become entangled, as predicted by quantum theory. On account of Wang’s new microscopy strategy, named quantum microscopy unintentionally (QMC), the ensnared particles are photons. On the whole, two snared photons are known as a biphoton, and, significantly for Wang’s microscopy, they act somehow or another as a solitary molecule that has twofold the energy of a solitary photon.
“UV light does not agree with cells. But if we can photograph the cell with 400-nanometer light and obtain the effect of 200-nm light, which is UV, the cells will be delighted, and we’ll have UV resolution.”
Lihong Wang, Bren Professor of Medical Engineering and Electrical Engineering,
Particles with larger moments have shorter wavelengths because, according to quantum mechanics, all particles are also waves and the wavelength of a wave is proportional to the momentum of the particle. Therefore, the wavelength of a biphoton is half that of a photon because it possesses twice the momentum of a photon.
This is crucial to QMC’s operation. The features of an object can only be imaged by a microscope if its minimum size is half the wavelength of light it uses. The microscope can see even smaller things because the wavelength of that light is shorter, which increases the resolution.
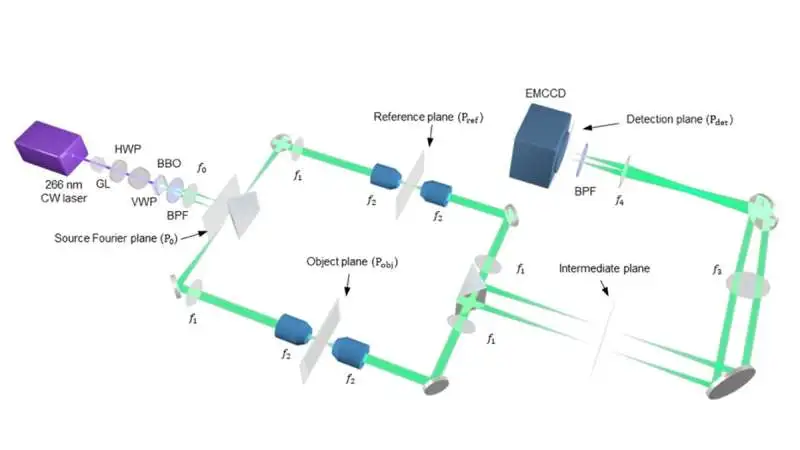
A schematic of the apparatus for coincidence-based quantum microscopy. Credit: Caltech
The wavelength of light used in a microscope can be reduced in a variety of ways, including Caltech Quantum Entanglement. For instance, purple light has a shorter wavelength than green light, and green light has a longer wavelength than red light. However, because of one more eccentricity of quantum physical science, light with more limited frequencies conveys more energy. In this way, when you get down to light with a frequency sufficiently low to picture small things, the light conveys such an excess of energy that it will harm the things being imaged, particularly living things like cells. Sunburns are caused by UV (ultraviolet) light, which has a short wavelength.
By employing biphotons that possess the shorter wavelength of higher-energy photons while carrying the lower energy of longer-wavelength photons, QMC circumvents this limitation.
According to Wang, “cells don’t like UV light.” Yet, on the off chance that we can utilize 400-nanometer light to picture the cell and accomplish the impact of 200-nm light, which is UV, the cells will be cheerful, and we’re getting the goal of UV.”
In order to accomplish this, Wang’s group constructed an optical device that directs laser light into a particular kind of crystal, converting some of the photons that pass through it into biphotons. The conversion is extremely rare and occurs in about one in a million photons, even when this special crystal is used. Utilizing a progression of mirrors, focal points, and crystals, each biphoton — which really comprises two discrete photons — is separated and transported along two ways, so one of the matched photons goes through the item being imaged and the other doesn’t.
The idler photon is the photon that does not pass through the object, while the signal photon does. These photons then proceed through additional optics until they arrive at a locator associated with a PC that forms a picture of the phone in view of the data conveyed by the sign photon. Amazingly, despite the object’s presence and their distinct paths, the paired photons remain entangled as a biphoton that behaves at half the wavelength.
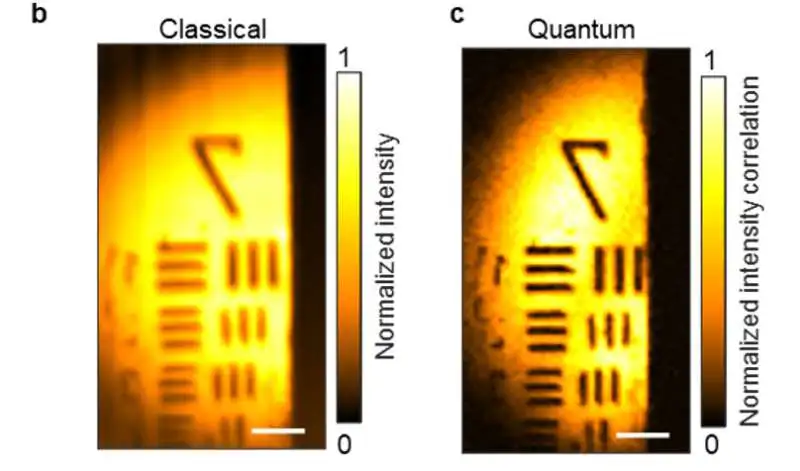
Pictures delivered by standard microscopy and quantum microscopy Credit: Caltech
Wang’s lab was not quick to deal with this sort of biphoton imaging, but it was quick to make a suitable framework utilizing the idea. “We developed a faster and more accurate entanglement measurement method in addition to what we believe to be a rigorous theory. We arrived at a tiny goal and imaged cells.”
Although there is no theoretical limit to the number of photons that can become entangled with one another, the momentum of the resulting multiphoton would be further reduced by each additional photon.
Although he points out that each additional photon further reduces the probability of a successful entanglement, which is already as low as a one-in-a-million chance, Wang claims that additional photon entanglement could be made possible through future research.
The work is described in the Nature Communications paper “Quantum Microscopy of Cells at the Heisenberg Limit.”
More information: Zhe He et al, Quantum microscopy of cells at the Heisenberg limit, Nature Communications (2023). DOI: 10.1038/s41467-023-38191-4