NASA’s retired Compton Gamma Ray Observatory (CGRO) mission has provided evidence of the existence of superheavy neutron stars. These are neutron stars with masses greater than 2.5 times the mass of the sun, and their existence was previously theoretical. The CGRO’s data showed gamma-ray bursts that were consistent with the collapse of massive stars into superheavy neutron stars. This discovery has important implications for our understanding of the evolution and fate of massive stars, as well as the structure and behavior of neutron stars.
Astronomers studying archival observations of powerful explosions known as short gamma-ray bursts (GRBs) have discovered light patterns that indicate the brief existence of a superheavy neutron star before it collapsed into a black hole. This ephemeral, massive object was most likely formed by the collision of two neutron stars.
“We looked for these signals in 700 short GRBs detected with NASA’s Neil Gehrels Swift Observatory, Fermi Gamma-ray Space Telescope, and Compton Gamma-ray Observatory,” said Cecilia Chirenti, a researcher at the University of Maryland, College Park (UMCP) and NASA’s Goddard Space Flight Center in Greenbelt, Maryland, who presented the findings at the American Astronomical Society’s 241st meeting in Seattle. “These gamma-ray patterns were discovered in two bursts observed by Compton in the early 1990s.”
A paper describing the results, led by Chirenti, was published in the scientific journal Nature.
When the core of a massive star runs out of fuel and collapses, a neutron star forms. This causes a shock wave, which blows the rest of the star away in a supernova explosion. Neutron stars typically pack more mass than our Sun into a ball the size of a city, but when their mass exceeds a certain threshold, they must collapse into black holes.
We know that short GRBs form when orbiting neutron stars crash together, and we know they eventually collapse into a black hole, but the precise sequence of events is not well understood.
Cole Miller
Both the Compton data and computer simulations revealed mega neutron stars that weighed 20% more than the most massive, precisely measured neutron star known, J0740+6620, which is nearly 2.1 times the mass of the Sun. Superheavy neutron stars are also nearly twice the size of ordinary neutron stars, or roughly twice the length of Manhattan Island.
The mega neutron stars spin nearly 78,000 times a minute – almost twice the speed of J1748-2446ad, the fastest pulsar on record. This rapid rotation briefly supports the objects against further collapse, allowing them to exist for just a few tenths of a second, after which they proceed to form a black hole faster than the blink of an eye.
“We know that short GRBs form when orbiting neutron stars crash together, and we know they eventually collapse into a black hole, but the precise sequence of events is not well understood,” said Cole Miller, a professor of astronomy at UMCP and a co-author of the paper. “At some point, the nascent black hole erupts with a jet of fast-moving particles that emits an intense flash of gamma rays, the highest-energy form of light, and we want to learn more about how that develops.”
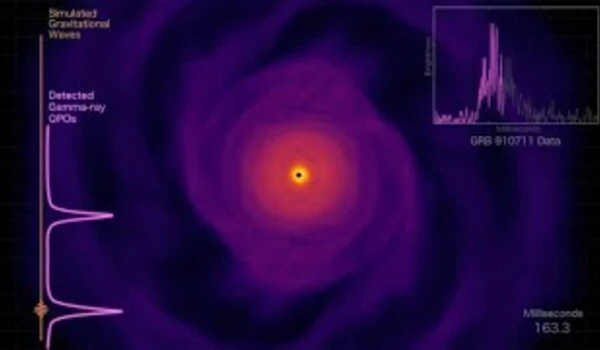
Short GRBs typically last less than two seconds but emit energy equivalent to that released by all the stars in our galaxy in one year. They can be seen from over a billion light-years away. Merging neutron stars also generate gravitational waves, which are ripples in spacetime that can be detected by an increasing number of ground-based observatories.
Computer simulations of these mergers show that gravitational waves have a sharp increase in frequency as the neutron stars merge, exceeding 1,000 hertz. These signals are too fast and too faint to be detected by existing gravitational wave observatories. However, Chirenti and her colleagues reasoned that similar signals could be seen in the gamma-ray emission of short GRBs.
These signals are known as quasiperiodic oscillations or QPOs for short. Unlike a tuning fork’s steady ringing, QPOs can be composed of several close frequencies that vary or dissipate over time. Both the gamma-ray and gravitational wave QPOs emerge from the swirling matter created by the merger of two neutron stars.
While no gamma-ray QPOs were observed in the Swift or Fermi bursts, two short GRBs recorded by Compton’s Burst And Transient Source Experiment (BATSE) on July 11, 1991 and November 1, 1993 fit the bill. The BATSE instrument’s larger area gave it an advantage in detecting these faint patterns — the tell-tale flickering that revealed the presence of mega neutron stars. The team rates the combined odds of these signals occurring by chance alone at less than 1 in 3 million.
“These results are very important as they set the stage for future measurements of hyper-massive neutron stars by gravitational wave observatories,” said Chryssa Kouveliotou, chair of the physics department at George Washington University in Washington, who was not involved in the work.
Gravitational wave detectors will be sensitive to kilohertz frequencies by the 2030s, providing new insights into the brief lives of super-sized neutron stars. Until then, the only tools for exploring them are sensitive gamma-ray observations and computer simulations.
Compton’s BATSE instrument was developed at NASA’s Marshall Space Flight Center in Huntsville, Alabama, and provided the first compelling evidence that gamma-ray bursts occurred far beyond our galaxy. The Compton Gamma Ray Observatory was deorbited and destroyed as it entered Earth’s atmosphere on June 4, 2000, after nearly nine years of operation.