As electronic, thermoelectric, and PC advancements have been scaled down to the nanometer scale, engineers have confronted a test concentrating on essential properties of the materials in question; generally speaking, targets are too small to possibly be seen with optical instruments.
Utilizing state-of-the-art electron magnifying lenses and novel methods, a group of specialists at the University of California, Irvine, the Massachusetts Institute of Technology, and different organizations have figured out how to plan phonons—vibrations in gem grids—in nuclear goals, empowering further comprehension of the manner in which intensity goes through quantum dabs, designed nanostructures in electronic parts.
“This research represents a significant step forward in the field because it is the first time we have been able to show direct evidence that the interplay between diffusive and specular reflection is heavily influenced by the atomistic structure.”
Xiaoqing Pan, UCI professor of materials science and engineering and physics,
To explore how phonons are dissipated by blemishes and connection points in gems, the scientists tested the unique way of behaving of phonons close to a solitary quantum dab of silicon-germanium involving vibrational electron energy misfortune spectroscopy in a transmission electron magnifying lens, gear housed in the Irvine Materials Research Institute on the UCI grounds. The consequences of the undertaking are the subject of a paper distributed today in Nature.
“We fostered an original method to differentially plan phonon momenta with nuclear goal, which empowers us to notice nonequilibrium phonons that exist close to the connection point,” said co-creator Xiaoqing Pan, UCI teacher of materials science and design and physical science, Henry Samueli Endowed Chair in Engineering, and IMRI chief. “This work denotes a serious step forward in the field since it’s whenever we first had the option to give direct proof that the transaction between diffusive and specular reflection generally relies upon the point-by-point atomistic design.”
As per Pan, at the nuclear scale, heat is shipped in strong materials as a rush of molecules dislodged from their harmony position as intensity creates some distance from the warm source. In gems, which have an arranged nuclear design, these waves are called phonons: wave bundles of nuclear removals that convey nuclear power equivalent to their recurrence of vibration.
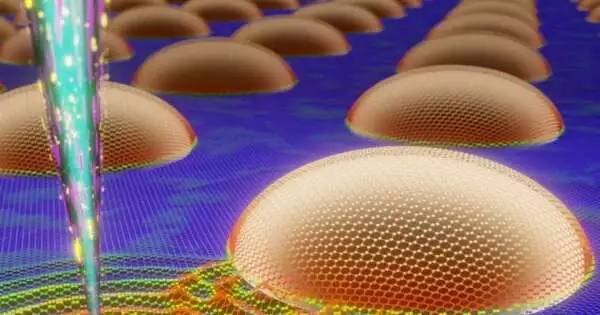
Utilizing a combination of silicon and germanium, the group had the option to concentrate on how phonons act in the confused climate of the quantum dab, in the connection point between the quantum spot and the encompassing silicon, and around the arch molded surface of the quantum dab nanostructure itself.
Xiaoqing Pan, UCI teacher of materials science and design and physical science, Henry Samueli Endowed Chair in Engineering, and head of the Irvine Materials Research Institute, is situated close to IMRI’s Nion Ultra Scanning Transmission Electron Microscope. The instrument was utilized to mention nuclear scale observable facts of phonon communications in gems, the subject of another paper in Nature. Photographer: Steven Zylius/UCI
“We found that the SiGe compound introduced a compositionally scattered structure that obstructed the productive proliferation of phonons,” said Pan. “Since silicon iotas are nearer together than germanium particles in their separate unadulterated designs, the combination extends the silicon molecules a piece.” Because of this strain, the UCI group found that phonons were being relaxed in the quantum dab because of the strain and alloying impact designed inside the nanostructure.
Container added that mellowed phonons have less energy, and that implies that each phonon conveys less intensity, diminishing warm conductivity accordingly. The conditioning of vibrations is behind one of the numerous instruments of how thermoelectric gadgets hinder the progression of intensity.
One of the vital results of the task was the improvement of another method for planning the heading of the warm transporters in the material. “This is practically equivalent to counting the number of phonons going up or down and taking the distinction, demonstrating their prevailing bearing of spread,” he said. “This method permitted us to plan the impression of phonons from interfaces.”
Hardware engineers have prevailed with regards to scaling down designs and parts in gadgets so much that they are presently down to a request for a billionth of a meter, a lot more modest than the frequency of noticeable light, so these designs are imperceptible to optical procedures.
“Progress in nanoengineering has dominated headways in electron microscopy and spectroscopy. However, with this examination, we are starting the method involved with making up for lost time,” said co-creator Chaitanya Gadre, an alumni understudy in Pan’s gathering at UCI.
A possible field to profit from this examination is thermoelectrics: material frameworks that convert intensity to power. Engineers of thermoelectric advancements try to plan materials that either block warm vehicles or advance the progression of charges, and iota-level information on how intensity is sent through solids implanted as they frequently are with deficiencies, deformities, and blemishes, will support this mission,” said co-creator Ruqian Wu, UCI teacher of physical science and stargazing.
“In excess of 70% of the energy delivered by human exercise is heat, so we should figure out how to reuse this back into a useable structure, ideally power to drive mankind’s rising energy demands,” Pan said.
More information: Chaitanya A. Gadre et al, Nanoscale imaging of phonon dynamics by electron microscopy, Nature (2022). DOI: 10.1038/s41586-022-04736-8