In a universe of materials that typically develop warming, one that therapists along one three-dimensional hub while extending along one more sticks out. That is particularly obvious when the surprising shrinkage is connected to a property significant for thermoelectric gadgets, which convert intensity to power or power to warmth.
In a paper just published in the journal Advanced Materials, a group of researchers from Northwestern University and the U.S. Branch of Energy’s Brookhaven National Laboratory depict the recently covered up sub-nanoscale starting points of both the surprising shrinkage and the extraordinary thermoelectric properties in this material, silver gallium telluride (AgGaTe2). The disclosure reveals a quantum mechanical twist in what drives the development of these properties—and opens up a completely new avenue for searching for new high-performance thermoelectrics.
The first creator of the paper, Hongyao Xie, a postdoctoral specialist at Northwestern University and the first creator of the paper, said that thermoelectric materials will be groundbreaking in green and maintainable energy advancements for heat energy harvesting and cooling — provided that their exhibit can be improved. “We need to find the hidden plan rules that will permit us to streamline the exhibition of these materials,” Xie said.
Thermoelectric gadgets are as of now utilized in restricted, specialty applications, including NASA’s Mars Wanderer, where intensity delivered by the radioactive rot of plutonium is changed into power. Future applications could incorporate materials constrained by voltage to accomplish truly stable temperatures, fundamental for the activity of innovative optical finders and lasers.
The primary barrier to more extensive reception is the requirement for materials with the perfect mix of properties, including great electrical conductivity and protection from the progression of intensity.
“The difficulty is, these helpful properties will more often than not contend,” said Mercouri Kanadzidis, the Northwestern teacher who started this review. “In many materials, electronic conductivity and warm conductivity are coupled and both are either high or low.” Not very many materials have an exceptional high-low blend.
Under specific circumstances, silver gallium telluride seems to have the perfect stuff — profoundly portable directing electrons and super low warm conductivity. As a matter of fact, its warm conductivity is fundamentally lower than hypothetical computations and examinations with comparative materials, for example, copper gallium telluride, would recommend.
The Northwestern researchers went to their partners and instruments at Brookhaven Lab to figure out why.
Brookhaven Lab physicist Emil Bozin, head of the primary investigation, said: “It took a fastidious X-beam assessment at Brookhaven’s National Synchrotron Light Source II (NSLS-II) to uncover a formerly covered up sub-nanoscale mutilation in the places of the silver particles in this material.”
Computational demonstrating uncovered how those mutilations trigger the one-hub gem shrinkage—and how that primary shift dissipates nuclear vibrations, along these lines, obstructing the spread of intensity in the material.
In any case, even with that comprehension, there was not a really obvious reason for what was driving the sub-nanoscale mutilations. The corresponding computational demonstration by Christopher Wolverton, a teacher at Northwestern, showed a novel and inconspicuous quantum mechanical beginning for the impact.
Together, the discoveries highlight another system for turning down warm conductivity and another core value in the quest for better thermoelectric materials.
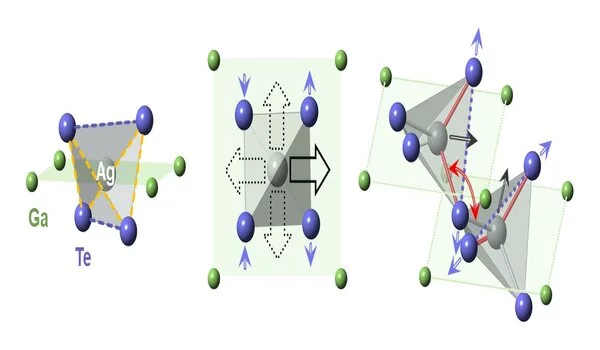
Mapping atomic positions
The group utilized x-beams at NSLS-II’s Pair Distribution Function (PDF) beamline to outline the “huge” scale plan of particles in both copper gallium telluride and silver gallium telluride over a range of temperatures to check whether they could find the reason why these two materials act in an unexpected way.
“A flood of hot air warms the example with degree-by-degree accuracy,” said Milinda Abeykoon, who is the lead researcher for the PDF beamline. “At every temperature, as the x-beams bob off the molecules, they produce designs that can be converted into high spatial resolution estimations of the distances between every particle and its neighbors (each pair). PCs then, at that point, collect the estimations into the most probable three-dimensional courses of action of the particles.
The team also performed additional calculations over a wider range of temperatures but at lower goals, using the light source at the Deutsches Elektronen-Synchrotron (DESY) in Hamburg, Germany.What’s more, they extrapolated their outcomes down to a temperature of outright zero, the coldest anything can get.
The information shows that the two materials have a jewel like tetragonal construction of corner-associated tetrahedra, one with a solitary copper molecule and the other with silver at the focal point of the three-dimensional article’s tetrahedral pit. Attributing what occurred as these diamondlike precious stones were warmed, Bozin said, “Promptly we saw a major distinction between the silver and copper forms of the material.”
The gem with copper at its center extended toward each path; however, the one containing silver extended along one hub while contracting along another.
“This peculiar way of behaving ended up having its starting point in the silver particles in this material having extremely huge plentifulness and cluttered vibrations inside primary layers,” said Simon Billinge, a teacher at Columbia University with a joint arrangement as a physicist at Brookhaven. “Those vibrations cause the connected tetrahedra to shake and hop with huge plentifulness,” he said.
“Thermoelectric materials will be transformational in green and sustainable energy technologies for heat energy harvesting and cooling—but only if their performance can be improved,”
Hongyao Xie, a postdoctoral researcher at Northwestern
This was a hint that the evenness—the customary game plan of particles—may be “broken” or disturbed at a more “nearby” (more limited size).
The group went to computational demonstrating to perceive how different nearby balance contortions of the silver particles would coordinate with their information.
“The one that worked the best showed that the silver molecule goes askew in the tetrahedron in one of four headings, close to the edge of the gem shaped by two of the tellurium iotas,” Bozin said. The irregular, topsy-turvy movement generally counterbalances, allowing the tetragonal balance to be maintained.
“However, we realize the bigger scope structure changes as well, by contracting in one course,” he noted. “It just so happens, the neighborhood and bigger scope twists are connected.”
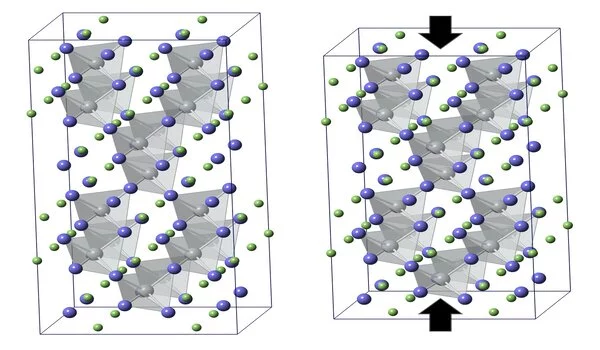
Twisting tetrahedrons
“The nearby mutilations are not totally arbitrary,” Bozin made sense of. “They are associated with adjoining silver molecules—those associated with a similar tellurium iota.” These neighborhood bends cause contiguous tetrahedra to pivot regarding each other, and that curving makes the precious stone grid recoil in one bearing. “
As the moving silver molecules bend the gem, they additionally disperse specific wavelike vibrations, called phonons, that permit intensity to be engendered through the cross section. Dissipating AgGaTe2’s energy-conveying phonons holds heat back from spreading, emphatically bringing down the material’s warm conductivity.
In any case, for what reason do the silver molecules move in any case?
The Brookhaven researchers had seen comparative conduct 10 years earlier, in a stone salt like lead-telluride material. All things considered, as the material was warmed, “solitary matches” of electrons were framed, producing little areas of parted electric charge called dipoles. Those dipoles pulled midway found lead particles askew and dissipated phonons.
However, in silver gallium telluride, there are no solitary matches. Thus, there should be something different in this material—and presumably other ‘diamondoid’ structures also, “Bozin said.”
Bending bonding behavior
Christopher Wolverton’s estimations at Northwestern uncovered that “something different” to be the holding attributes of the electrons circling the silver particles.
“Those estimations analyzed the silver and copper particles and observed that there is a distinction in the course of action of electrons in the orbitals with the end goal that silver tends to frame more fragile bonds than copper,” said Northwestern’s Xie. “Silver needs to bond with fewer adjoining tellurium particles; it needs a more straightforward holding climate.”
Rather than restricting similarly with each of the four surrounding tellurium molecules, as copper does, silver preferentially (albeit haphazardly) draws nearer to two of the four. Those holding electrons pull the silver iota askew, setting off the curving, shrinkage, and vibrational changes that at last lower the warm conductivity in AgGaTe2.
“We’ve stumbled upon a new mechanism by which lattice thermal conductivity can be reduced,” Northwestern’s Mercouri Kanadzidis said. “Perhaps this mechanism can be used to engineer, or look for, other new materials that have this type of behavior for future high-performance thermoelectrics.”