Researchers created and simulated the first topological acoustic transistors, which use sound waves instead of electrons, and proposed a connection architecture to form a universal logic gate that can turn the flow of sound on and off.
Future transistors that use far less energy than current devices may rely on exotic materials known as “topological insulators,” in which electricity flows only across surfaces and edges with virtually no energy dissipation. Harvard scientists have invented and simulated the first acoustic topological transistors, which operate with sound waves rather than electrons, in research that could pave the way for such electronic topological transistors.
Topological materials conduct electrons without loss along their surface and edges, making them promising materials for dissipation-free, high-efficiency electronics. Researchers are particularly interested in using these materials as transistors, which serve as the foundation of all modern electronics. However, there is a problem: while transistors turn electronic current on and off, it is difficult to turn off the dissipationless flow of electrons in topological materials.
Topologically protected acoustic transport control has applications in a variety of important fields, including efficient acoustic-noise reduction, one-way acoustic propagation, ultrasound imaging, echolocation, acoustic cloaking, and acoustic communications.
Harris Pirie
Harvard University researchers have designed and simulated the first topological acoustic transistors, which use sound waves instead of electrons, and proposed a connection architecture to form a universal logic gate that can turn the flow of sound on and off.
“There has been a lot of interest in developing a topological electronic transistor since the advent of topological materials around 2007,” said Jenny Hoffman, Clowes Professor of Science at the Harvard John A. Paulson School of Engineering and Applied Sciences (SEAS) and the Department of Physics. “Although the materials we used will not produce an electronic topological transistor, our general design process applies to both quantum materials and photonic crystals, raising hopes that electronic and optical equivalents will be available soon.”
The findings were published in Physical Review Letters. The researchers were able to avoid the complicated quantum mechanics of electron topological insulators by using acoustic topological insulators.
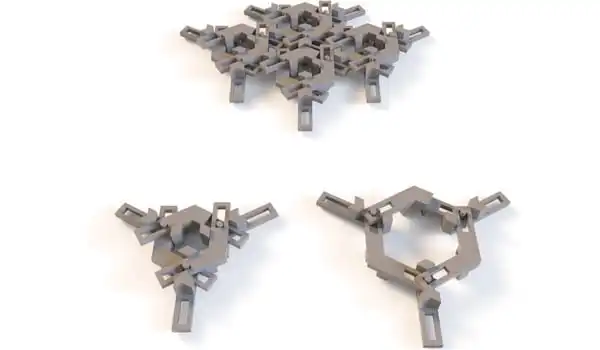
“Because the equations for sound waves are exactly solvable, we were able to numerically find just the right combination of materials to design a topological acoustic waveguide that turns on when heated and off when cooled,” said Harris Pirie, the paper’s first author and a former graduate student in the Department of Physics.
Pirie is a Marie Curie Postdoctoral Fellow at Oxford University right now.
Topology is a branch of mathematics that studies the nature of shapes that are not deformed. For example, a doughnut-shaped object can be deformed into the shape of a mug, so that the hole in the doughnut becomes the hole in the cup’s handle. The object, on the other hand, couldn’t lose the hole without changing fundamentally.
The researchers created an airtight box with a honeycomb lattice of steel pillars anchored to a high-thermal-expansion plate. The lattice is divided into two halves, with slightly larger pillars on one half and slightly smaller pillars on the other. The size and spacing of the pillars determine the topology of the lattice, which determines whether or not sound waves can travel along a designated channel. The researchers then designed a second device that converts ultrasound into heat.
Heat causes the pillar lattice to expand, altering the waveguide’s topology. When these two devices are linked together, the output of one waveguide can control the state of the next, just as electrons flowing in a conventional transistor can toggle other transistors.
These acoustic topological switches are scalable, which means that the same design that works with ultrasonic frequencies at the centimeter scale could also work at sub-millimeter sizes and frequencies commonly used to transmit surface acoustic waves, potentially helping to overcome limitations in integrated phononic circuits.
“Topologically protected acoustic transport control has applications in a variety of important fields, including efficient acoustic-noise reduction, one-way acoustic propagation, ultrasound imaging, echolocation, acoustic cloaking, and acoustic communications,” said Pirie. These acoustic metamaterials could also be used in education.
“Acoustic metamaterials, in contrast to quantum-mechanical systems, are straightforward, tangible, and intuitive. They provide an accessible introduction to cutting-edge topics in condensed matter physics such as topological insulators “Hoffman stated. The team intends to create a public demonstration of these devices, which students and museum visitors will be able to touch, toggle, and hear.