Semiconductors are shifting away from rigid substrates that are cut or formed into thin discs or wafers and toward more flexible plastic materials and even paper as a result of new material and fabrication discoveries. The trend toward more flexible substrates has resulted in the fabrication of a wide range of devices, from light-emitting diodes to solar cells and transistors.
Georgia Tech researchers developed a material that acts as a second skin layer and is up to 200 percent stretchable than its original dimension without significantly losing its electric current. According to the researchers, soft flexible photodetectors could improve the utility of medical wearable sensors and implantable devices, among other applications.
Over a three-year period, Georgia Tech researchers from both mechanical and computing engineering labs collaborated to demonstrate a new level of stretchability for a photodetector, a device made of a synthetic polymer and an elastomer that absorbs light to produce an electrical current.
Photodetectors are now used in wearable health monitoring devices such as rigid fingertip pulse oximeter reading devices. They are commonly used on wearable electronics to convert light signals to electrical signals.
We’ve shown that you can build stretchability into semiconductors that retains the electrical performance needed to detect light levels that are around hundred million times fainter than produced by a light bulb used for indoor illumination.
Canek Fuentes-Hernandez
Stretchable like a Rubber Band
Given that conventional flexible semiconductors fail at a few percentage points of strain, the Georgia Tech findings are “an order of magnitude improvement,” according to Olivier Pierron, professor in the George W. Woodruff School of Mechanical Engineering, whose lab measures the mechanical properties and reliability of flexible electronics under extreme conditions.
“Think of a rubber band or something that’s soft and stretchable like human skin but has similar electrical semiconducting properties to solid or rigid semiconductors,” said Canek Fuentes-Hernandez, a co-PI who previously worked in the School of Electrical and Computer Engineering (ECE) and is now an associate professor in Electrical and Computer Engineering at Northeastern University in Boston.
“We’ve shown that you can build stretchability into semiconductors that retains the electrical performance needed to detect light levels that are around hundred million times fainter than produced by a light bulb used for indoor illumination,” he said.
Extraordinary Tenacity and Teamwork
Youngrak Park, the study’s first author and a Ph.D. candidate in ECE, was overseen by Bernard Kippelen, vice provost for International Initiatives and an ECE professor. Park discovered the right combination of chemical compounds that produced a super-soft material with the ability to generate and conduct electricity when exposed to light after two and a half years of research.
Park discovered the ideal ratio for all components of the semiconductor layer in order to maintain high performance in the photodetector. However, proving the materials’ stretchability was difficult, especially given that a single layer was 1,000 times thinner than a human hair.
Park enlisted the help of Kyungjin Kim, a Ph.D. mechanical engineering student at Georgia Tech at the time, to test the material’s dependability. He kept giving Kim bigger, thicker samples until one with a thickness of 500 nanometers worked.
“It was still extremely thin. It would simply crumble in dry conditions. To keep its shape, we had to use a water reservoir “Kim, who is now an assistant professor in the Department of Mechanical Engineering at the University of Connecticut, recalled.
Pierron elaborated on how difficult it was to measure the pure mechanical properties of a photoactive layer, saying, “Electronic devices are typically very brittle, which is fine for conventional devices fabricated on rigid substrates. However, when soft substrates are used, this becomes an issue.”
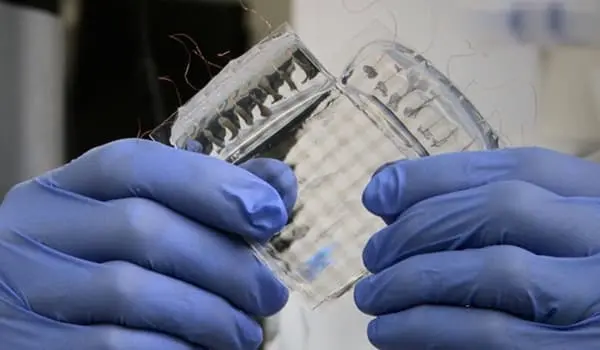
The water acted like plastic wrap, holding the thin films in place without crumbling or losing shape, allowing the researchers to stretch and measure the material’s mechanical properties. Electronic terminals had to be embedded on the device to test for electrical signals coming out of it under illumination. Those terminals, however, had to be deformable as well, or the entire device would become rigid.
“Fabricating stretchable electronic terminals was a significant challenge in and of itself,” said Felipe Andres Larrain, an ECE PhD graduate who worked closely with Park and focused on the embedded components. He is now an assistant professor at Chile’s Adolfo Ibáez University.
While this ground-breaking material was initially integrated into a photodetector and tested for electrical functionality, more testing and optimization are required to demonstrate the material’s stretchability under multimodal loads and shelf stability.
“What excites me is what these materials and devices will allow us to create. Specifically, the concept of intelligent systems. You have functional surfaces that incorporate sensors that monitor a wide range of physical properties “Graham, former chair of the Woodruff School of Mechanical Engineering and current Dean of Engineering at the University of Maryland, made the statement.
“This is an excellent example of interdisciplinary research; none of this would have been possible without the collaboration of electrical and mechanical engineers,” Kippelen said. “We had no prior experience with stretchable materials in the lab. It took a lot of perseverance, creativity, and hard work to figure out how to measure this.”
New Smart Applications Possible
The researchers are most excited about the material’s potential to improve medical wearables. Wristwatches that use rigid biosensors typically have limitations because flexing the wrist completely changes the sensor’s measurements. When a person moves, they are subject to “motion artifact,” or degraded image quality.
“Moving around can drastically affect the usability of collected data, but being able to reposition devices on the body to minimize or eliminate motion artifact is a big deal,” said Gabriel Cahn, project manager for Huxley Medical, an Atlanta-based biosensor startup that recently graduated from Georgia Tech with a doctorate in flexible electronics. “Having electronics that can flex, twist, bend, and conform to non-flat surfaces while also moving with your body allows you to place these sensors in more advantageous locations to collect biometric data. It will be far more useful in assisting in the diagnosis or monitoring of existing medical illnesses.”
Beyond wearables for health monitoring, the research team sees a wide range of applications for the soft and stretchable polymer blend. “The soft device could also be appealing for implantable electronics for bio-electronic applications because the interfaces comply with the dynamic motion of soft biological tissues, reducing foreign body reaction,” Kim said.
“The potential is fantastic,” Larrain added. “In the long run, you could create sensors that could augment or even replace the human eye, or you could apply them to robotic eyes.”
Fuentes envisions the material being used in smart agriculture applications, where farmers could embed light sensors in fruits and other produce to monitor growth, disease, and harvesting timing. Kippelen believes that the rubber-like photodiodes that detect ultralow light levels could be used to detect, identify, and characterize ionizing radiation for nuclear fuel cycle monitoring.