Some major scientific breakthroughs are never made. They have been borrowed. That’s what happened when scientists sought the help of proteins sourced from an odd source: green algae. Chlamydomonas reinhardtii cells are adorned with proteins that can detect light. That talent, discovered in 2002, instantly piqued the interest of brain scientists. A light-sensing protein promises the ability to control neurons — the brain’s nerve cells — by turning them on and off at precisely the correct place and right time.
Nerve cells that have been genetically modified to synthesize algal proteins are transformed into light-controlled puppets. A burst of light could cause a dormant neuron to fire messages or make an active cell dormant.
“This molecule is the light sensor that we needed,” says vision neuroscientist Zhuo-Hua Pan, who had been searching for a way to control vision cells in mice’s retinas.
Because of the coupling of light (opto) and genes, the technology allowed by these loaner proteins is now known as optogenetics. Opogenetics has yielded significant insights into how memories are preserved, what causes perceptions, and what goes wrong in the brain during depression and addiction in less than two decades.
Scientists have experimented with mouse hallucinations by using light to stimulate the activity of specific nerve cells: mice have seen lines that aren’t there and remembered a room they’ve never been in. Opogenetics has been used by scientists to make mice fight, mate, and eat, as well as to give blind mice sight. Opogenetics has restored elements of a blind man’s vision in a significant first.
Nerve cells that have been genetically modified to synthesize algal proteins are transformed into light-controlled puppets. A burst of light could cause a dormant neuron to fire messages or make an active cell dormant. This molecule is the light sensor that we needed.
Zhuo-Hua Pan
An early indication of optogenetics’ potential arrived at 1 a.m. on August 4, 2004. Ed Boyden, a neuroscientist at Stanford, was checking on a dish of neurons that had a gene for one of the algal light sensors called channelrhodopsin-2. Boyden planned to flash blue light at the cells to check if they sent out signals. Boyden noted in a 2011 account that the very first cell he checked responded to the light with a surge of activity. The potential sparked by that small spark of activity, as documented in a technical paper published in 2005 by Boyden, Karl Deisseroth of Stanford University, and colleagues, swiftly became reality.
In Pan’s lab, light-responsive proteins restored vision in mice with damaged retinas, a finding that has now led to a clinical trial in people. Optogenetics’ promise wasn’t a given in those early days, as scientists were first learning how to use these proteins in neurons. “At that time, no one anticipated that this optogenetic work would have such a huge impact,” Pan says.
Since those early discoveries, the algae’s light sensors have been used in a variety of brain research fields. Talia Lerner, a neuroscientist at Northwestern University in Chicago, for example, utilizes optogenetics to explore cell connections in the mouse brain. The technique enables her to dissect the interactions between cells that create and respond to dopamine, a chemical messenger involved in movement and reward. These cellular connections, highlighted by optogenetics, may aid in the discovery of subtleties about motivation and learning. “Without optogenetics, my research would not be conceivable in its current form,” she explains.
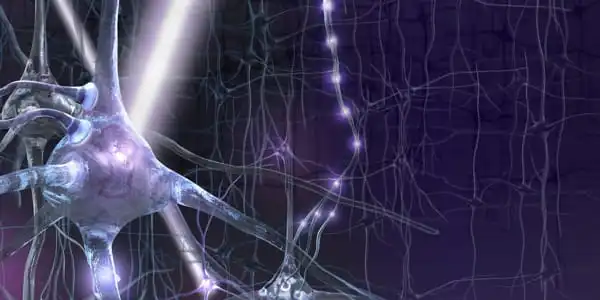
Optogenetics is also essential for Jeanne Paz of the Gladstone Institutes in San Francisco. She and her colleagues have been searching for cells that can prevent seizures from spreading across the brain. Opogenetics is vital to her investigation since it allows her to regulate different groups of neurons. “We truly couldn’t pose these questions with any other instrument,” Paz explains.
Paz’s optogenetics-assisted search led her to the thalamus, a brain region that serves as a hub for many neuronal networks. “I recall the goose bumps I got the first time I shined the laser into the thalamus and it stopped the seizure,” she says.
So far, optogenetics research has primarily been conducted in mice. However, Columbia University’s Yasmine El-Shamayleh believes that insights into more sophisticated brains, such as those of primates, will be discovered shortly. Boyden and colleagues described optogenetics in a macaque in 2009. El-Shamayleh and others are pushing hard for this field of research. “We are clearly on the edge” of discovering some fascinating primate brain concepts, such as how the brain converts signals from the sight into perceptions, she says.
Optogenetics has advanced rapidly. Scientists have developed and optimized novel light sensors, as well as new methods for merging them with other techniques. According to Lerner, the early spirit of sharing among optogenetics pioneers was a significant reason for today’s widespread innovation. Deisseroth would hold workshops at Stanford on a regular basis to instruct other scientists on the approach. Lerner believes that “in some respects, that’s as essential as inventing it.”
So it’s worth pausing for a moment to thank the initial sharers. Whatever happens next in this fast-moving field, one thing is certain: brain scientists will be eternally grateful to algae.