The morphology, chemical properties, and mechanical properties of cancerous cells and normal cells clearly differ. The pathological processes of human cells and tissues can be better understood by analyzing the cytochemical and mechanical properties of tumor tissues.
Among the current recognition techniques for the morphology, mechanics, and synthetic properties of tissue and cells, confocal Raman spectroscopy can distinguish the substance properties of miniature areas of tests without contact or name, and confocal Brillouin spectroscopy can identify the mechanical properties of miniature districts of tests without contact or name.
It is anticipated that a new method for the detection of multi-dimensional pathological information in tissue and cells will be created by combining confocal Raman spectroscopy with Brillouin spectroscopy simultaneously and in-situ to detect the three-dimensional morphology, chemical properties, and mechanical properties of micro-regions of tissues and even subcellular structures.
The current confocal Raman/Brillouin spectroscopic microscopic imaging technology lacks high-precision real-time focusing, limiting the theoretical spatial resolution of a confocal spectrum microscopy system by altering the size of the spot focused on the sample as the sample changes during the scanning process.
Second, the confocal spectrum microscope is susceptible to system drift because of its weak Raman and Brillouin scattering spectra and long integration time. This causes defocus, which lowers imaging quality and spatial resolution. Additionally, the vertical incidence fluorescence signal decreased the sample’s Raman spectrum’s signal-to-noise ratio when used for biological tissue slice sample imaging. As a result, the accuracy of Raman and Brillouin spectrum detection is impacted and decreased.
A group of researchers from Beijing Institute of Technology, led by Professor Weiqian Zhao, proposed a brand-new divided-aperture laser differential confocal Raman-Brillouin spectrum microscopy (DLDCRBSM) that is capable of high stability, high resolution, and anti-scattering in a recent paper that was published in the journal Light Science & Application.
It used divided-aperture technology to effectively suppress the interference of the stray light of the defocus layer and the reflected lights to improve the signal-to-noise ratio, and it used coaxial exciting and high-resolution separate detection to achieve high-stability and high-spatial-resolution spectrum simultaneous imaging for the geometrical topography, Raman, and Brillion spectroscopy from the same region. It combined divided-aperture laser differential confocal microscopy with Raman
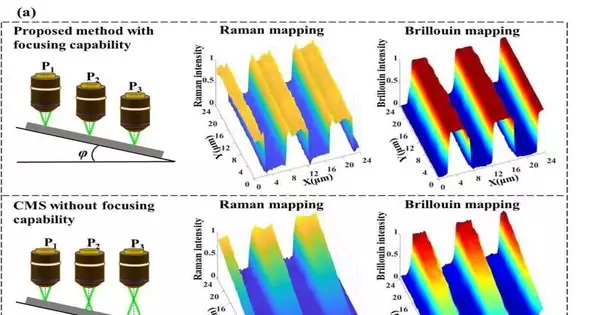
(a) When the sample is tilted, the DLDCRBSM and confocal Raman system (CRM) test results The mapping results of DLDCRBSM are clear (upper) when focusing capability is present. The traditional CRM/Confocal Brillouin Microscope’s mapping results are ambiguous (lower) without the ability to focus. b) The DLDCRBSM compresses the size of the focal region in the axial direction in comparison to the conventional CRM because it spatially separates the Raman spectrum’s excitation and collection light paths. As a result, the system is able to prevent stray light from interfering with the defocusing layer. Credit: The divided-aperture differential confocal Raman-Brillouin spectrum microscope with high spatial resolution and 3D imaging focusing and tracking capability is developed on the basis of the proposed method by Lirong Qiu, Yunhao Su, Ke-Mi Xu, Han Cui, Dezhi Zheng, Yuanmin Zhu, Lin Li, Fang Li, and Weiqian Zhao. It has a Raman spectrum detection resolution of 0.7 cm1, a Brillouin spectrum detection resolution of 0.5 GHz, a spectral image lateral resolution of greater than 400nm, and an axial focusing accuracy of 1nm.
The laid-out magnifying instrument was utilized for a strip-molded polymethyl methacrylate (PMMA) test on Si substrate and got the unmistakable pictures of the test because of its pivotal constant centering. Thus, it checked that the proposed strategy has the counter-float ability. The magnifying instrument was utilized for the straightforward two-layer example with the upper layer of PMMA and the lower layer of SiO2, and the estimations show that the magnifying lens has the capacity of smothering the obstruction of defocused stray light.
Raman and Brillouin planning of gastric malignant growth tissues and neighboring typical tissues was done by utilizing the newly created differential confocal spectroscopy magnifying instrument. The outcomes affirmed the past speculation that the progression of protein substances in diseased tissues and the progression of tissue viscoelasticity lead to an increment in obtrusiveness.
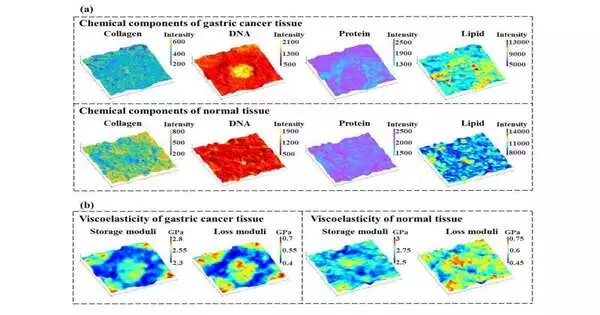
Fig. 3. (a) The three-dimensional distribution map of various chemical components in the scanned area of normal and gastric cancer tissue b) The three-dimensional distribution map of loss modulus and storage modulus in the scanned area of normal tissue surrounding the cancer and gastric cancer tissue The recurrence shift and full width at the half limit of the Brillouin range address the capacity modulus (flexibility) and misfortune modulus (consistency) of a substance separately. Credit: Lirong Qiu, Yunhao Su, Ke-Mi Xu, Han Cui, Dezhi Zheng, Yuanmin Zhu, Lin Li, Fang Li, and Weiqian Zhao present the chemical imaging results of gastric cancer tissues and the normal tissues that are adjacent to them using this differential confocal spectroscopy microscope in Figure 3(a). The concentration is identified by the intensity of the distinctive peak of the Raman spectra.
The distribution of collagen in gastric cancer tissues was distinct and low in comparison to the normal tissues that were adjacent. The distribution of DNA material in gastric cancer cells is extensive and has a high concentration. The cell matrix of gastric cancer tissues contained little protein. While the distribution of lipids in healthy tissue is relatively uniform, gastric cancer tissue has a high lipid concentration within the matrix.
Figure 3(b) shows the imaging aftereffects of the mechanical properties of the gastric malignant growth tissue and adjoining ordinary tissues by the differential confocal spectroscopy magnifying instrument. A substance’s energy storage modulus, or elasticity, is depicted by its frequency shift in the Brillouin spectrum, while its loss modulus, or viscosity, is depicted by its full width at half maximum. The elasticity of gastric cancer cells and their intercellular substance was lower, and the elasticity of the cancer cell nucleus was higher, when compared to the results of mechanical imaging of adjacent normal tissues. The interstitial tissue and gastric cancer cells were less sticky, while the cancer cell nucleus was more sticky.
In this study, a laser differential confocal Raman-Brillouin spectroscopy imaging method with high stability, high resolution, and anti-scattering was proposed. The corresponding instrument was successfully developed to detect the three-dimensional morphology, mechanical properties, and multi-dimensional information of the samples, and its application was verified through the characterization analysis of tumor tissues. The study of the cancer process and its treatment may benefit from this novel approach.
More information: Lirong Qiu et al, A high-precision multi-dimensional microspectroscopic technique for morphological and properties analysis of cancer cell, Light: Science & Applications (2023). DOI: 10.1038/s41377-023-01153-y