The Rydberg state is common in atoms, molecules, and solids, among other physical platforms. In particular, Rydberg excitons are electron-hole pair, highly excited Coulomb-bound states that were first discovered in the 1950s in the semiconductor material Cu2O.
Rydberg moiré excitons, which are moiré-trapped Rydberg excitons in the monolayer semiconductor WSe2 adjacent to small-angle twisted bilayer graphene (TBG), were observed by Dr. Xu Yang and his colleagues from the Institute of Physics of the Chinese Academy of Sciences (CAS) in collaboration with researchers led by Dr. Yuan Shengjun of Wuhan University in a study that was recently published in Science.
A wide range of applications in sensing, quantum optics, and quantum simulation are possible thanks to Rydberg excitons’ solid-state nature, large dipole moments, strong mutual interactions, and greatly enhanced interactions with the surrounding environment.
However, Rydberg excitons’ potential has not been fully utilized by researchers. The difficulty of effectively trapping and manipulating Rydberg excitons is one of the main obstacles. A possible path forward is provided by the development of two-dimensional (2D) moiré superlattices with highly tunable periodic potentials.
Dr. Xu Yang and his colleagues have been investigating the use of Rydberg excitons in 2D semiconducting transition metal dichalcogenides like WSe2 in recent years. Exotic phases in a nearby 2D electronic system can be detected using a new Rydberg sensing method that takes advantage of the sensitivity of Rydberg excitons to the dielectric environment.
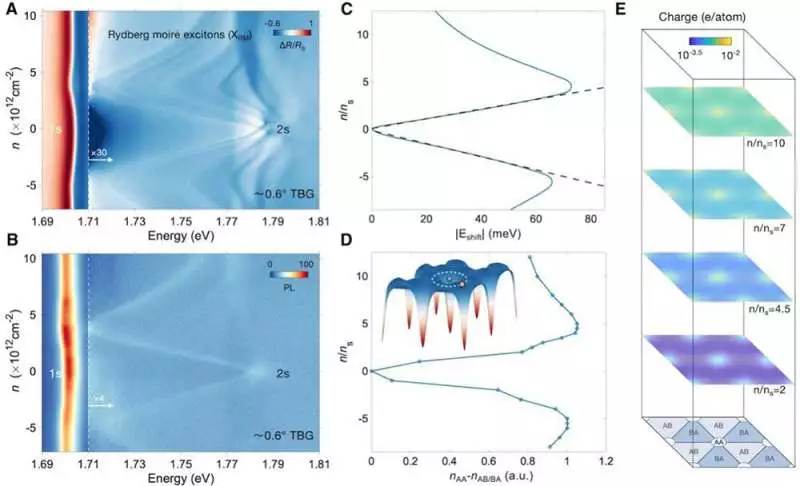
Numerical calculations of the spatial charge distribution in TBG at various doping levels and spectroscopic evidence of the Rydberg moiré exciton formation in WSe2 near 0.6° TBG. Credit: IOP
In this review, utilizing low-temperature optical spectroscopy estimations, the scientists previously found the Rydberg moiré excitons appearing as different energy splitting’s, an articulated red shift, and a limited linewidth in the reflectance spectra.
The researchers attributed these observations to the spatially varying charge distribution in TBG, which produces a periodic potential landscape—the so-called moiré potential—for interacting with Rydberg excitons through numerical calculations carried out by the Wuhan University group.
Due to the spatially accumulated charges centered in the AA-stacked regions of TBG, the constituent electron and hole of a Rydberg exciton interact largely unequally between layers, which results in the strong confinement of Rydberg excitons. As a result, the Rydberg moiré excitons perform electron-hole separation and have the characteristics of excitons that transfer charge for a long time.
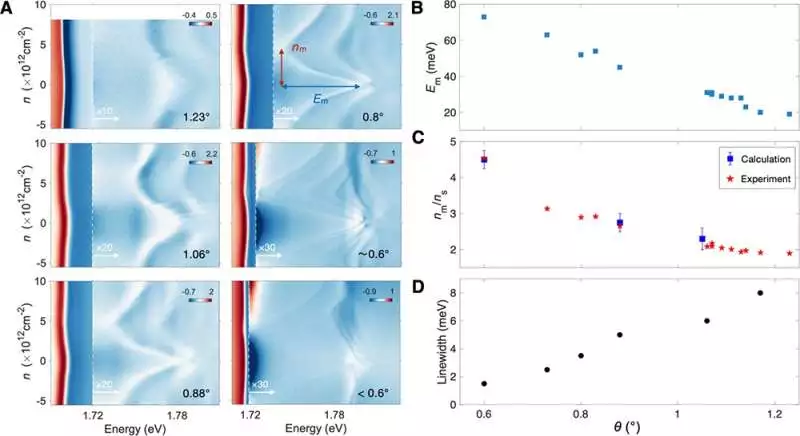
Crossover to the strong-coupling regime and twist angle dependences. Credit: IOP
The specialists showed a clever strategy for controlling Rydberg excitons, which is challenging to accomplish in mass semiconductors. The optical lattices created by a standing-wave laser beam or arrays of optical tweezers utilized for Rydberg atom trapping are analogous to this study’s long-wavelength moiré superlattice (tens of nm).
In addition, the system is highly controllable thanks to tunable moiré wavelengths, in-situ electrostatic gating, and a longer lifetime. A strong light-matter interaction facilitates easy optical excitation and readout.
With potential applications in quantum information processing and computation, this study may present new opportunities for realizing the next step in Rydberg-Rydberg interactions and coherent control of Rydberg states.
More information: Qianying Hu et al, Observation of Rydberg moiré excitons, Science (2023). DOI: 10.1126/science.adh1506