Quantum PCs process data utilizing quantum bits, or qubits, in light of delicate, brief quantum mechanical states. To make qubits powerful and tailor them for applications, scientists from the Branch of Energy’s Oak Edge Public Lab tried to make another material framework.
“We are seeking another course to make quantum PCs utilizing novel materials,” said ORNL materials researcher Robert Moore, who co-droved a review distributed in Cutting Edge Materials with ORNL partner Matthew Brahlek, who is likewise a materials researcher.
They coupled a superconductor, which offers no protection from electrical flow, with a topological cover that has electrically conductive surfaces yet is protected inside. The outcome is a molecularly sharp connection point between glasslike dainty movies with various symmetric courses of action of particles. The original connection point that they planned and designed may lead to fascinating physical science and host an exceptional quantum building block with potential as an unrivaled qubit.
“The thought is to make qubits with materials that have more hearty quantum mechanical properties,” Moore said. “What is significant is that we have figured out how to control the electronic construction of the topological protector and the superconductor autonomously, so we can tailor the electronic design at that connection point. This had never been finished.”
Controlling the electronic construction on the two sides of a point of interaction might make what many refer to as Majorana particles inside the material. “In nature, we have particles and antiparticles, for instance, electrons and positrons, which obliterate each other when they come into contact. A Majorana molecule is its own antiparticle,” Moore said. In 1937, Ettore Majorana anticipated the presence of these fascinating particles, whose presence still needs to be demonstrated.
In 2008, theoretical physicists Liang Fu and Charlie Kane of the College of Pennsylvania suggested that making an original connection point between a topological cover and a superconductor would create topological superconductivity, another period of issue anticipated to have Majorana particles.
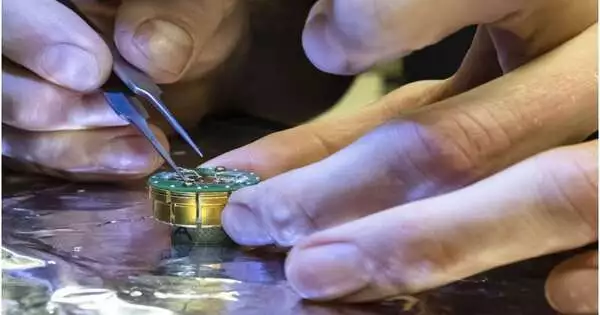
ORNL’s Matt Brahlek utilized sub-atomic shaft epitaxy to grow a slim film connection point of topologically protecting and superconducting materials, molecule by iota. Credit: Carlos Jones/ORNL, U.S. Dept. of Energy
“In the event that you have a couple of Majorana particles and move them around one another, there is a memory of this movement. They generally know one another’s area,” Moore said. “This cycle could be utilized to encode quantum data and register in new ways.”
In any case, acknowledgment of another period of issue that can have Majorana particles relies upon viewing it as the right material. Such an accomplishment takes a different group of specialists.
At the point when Moore came to ORNL in 2019, he got another mastery point: settled photoemission spectroscopy, or ARPES, a procedure for examining the electronic design of materials. ARPES depends on the photoelectric impact, for which Albert Einstein was granted the 1921 Nobel Prize in physical science. It shines a light source on an example and describes electrons catapulted from the material surface when the electrons retain energy from the photons. The strategy assists researchers with understanding how electrons act inside a material.
This essential interest in ARPES ability assisted ORNL in winning its offer to lead one of five DOE Public Quantum Data Science Exploration Focuses, the Quantum Science Center, which kicked off in 2020. Driven by ORNL’s Travis Unassuming, the QSC plans to acknowledge quantum processing and detection applications by creating equipment and calculations and finding novel materials. Moore and his associates center around topological materials for equipment advancement. Since April, Moore has additionally co-coordinated ORNL’s Interconnected Science Biological System, or Meet, with Ben Mintz to foster labs representing things to come—savvy, independently controlled cycles and analyses with the possibility to alter research results.
Brahlek, who joined ORNL in 2018 and has, as of late, gotten a DOE Early Vocation Exploration Grant, is a specialist in the accurate blend of materials. To make superclean interfaces between a superconductor and a topological cover, he utilized sub-atomic bar epitaxy, a technique the industry utilizes for the enormous-scope manufacture of semiconductors for electronic gadgets.
With assistance from previous postdoctoral individual Tyler Smith, Brahlek played out the combination under ultrahigh vacuum. “Inside the chamber, there are fewer atoms skipping around than in space. It is a truly perfect climate. It should be all around controlled,” Brahlek said. “You start with little heaters, each containing one component. Every heater warms until the component inside begins to sublimate, or pass from a strong to a fume state. This produces light emissions. They all combine on a gem substrate and stick.”
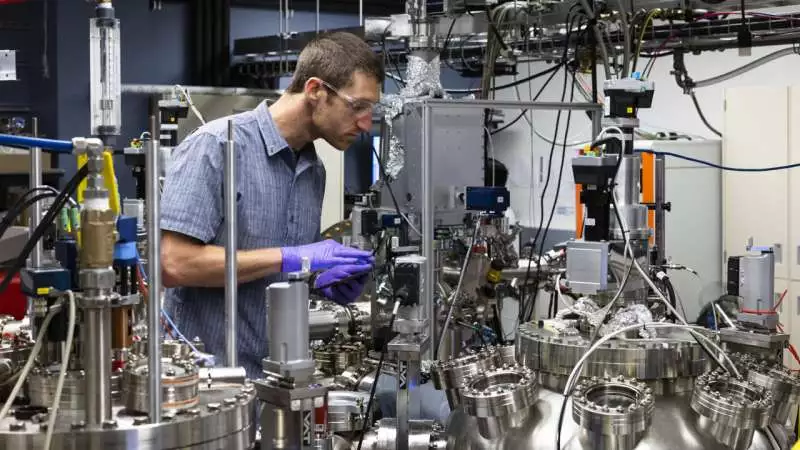
Associating wires to the point of interaction of the topological protector and superconductor empowers the examination of novel electronic properties. Specialists go for the gold on hypothesized Majorana particles. Credit: Carlos Jones/ORNL, U.S. Dept. of Energy
He co-saved iron, selenium, and tellurium to make a superconductor that was one nuclear layer thick. “In the event that you can get the circumstances precisely on, the stored iotas will synthetically bond and collect, nuclear layer by nuclear layer, into a glasslike slender film,” Brahlek said.
“A vital aspect for obtaining the outcomes was understanding how to join bismuth telluride with iron selenide telluride at a nuclear point of interaction to acquire the ideal electronic way of behaving,” Brahlek said.
That achievement was precarious on the grounds that the superconductor’s cross section of iron, selenium, and tellurium involves requested square cells, though the topological protector is an organization of bordering triangles. “We’re putting something square on something three-sided, yet shockingly, the translucent film develops pleasantly,” Brahlek said. “This achievement requires understanding the physical science and science that occur at these connection points, which is basic to consolidating topological and superconducting properties in a solitary stage.”
That stage is the topological superconductor. To comprehend its topological properties, Moore utilized turn-settled ARPES, with assistance from ORNL postdoctoral individual Qiangsheng Lu, to test quantum turn-subordinate electronic construction at the point of interaction between the topological encasing and the superconductor. In the mean time, to affirm its superconducting conductivity, Brahlek and previous ORNL postdoctoral colleagues Yun-Yi Pai and Michael Chilcote helped with estimations of electrical obstruction.
“We had the option to perceive how the different electronic designs were connecting at the connection point, and we had the option to control those communications to guarantee every one of the elements for topological superconductivity exist,” Moore said. “We found that the ideal topological properties just exist for explicit selenium doping ranges. This was unexpected and urgent for making qubits.”
In the meantime, Hoyeon Jeon and An-Ping Li at ORNL’s Middle for Nanophase Materials Sciences utilized examining burrowing microscopy to portray jumble in the materials. ORNL staff researchers Hu Miao and Satoshi Okamoto gave trial and hypothetical directions all through the review.
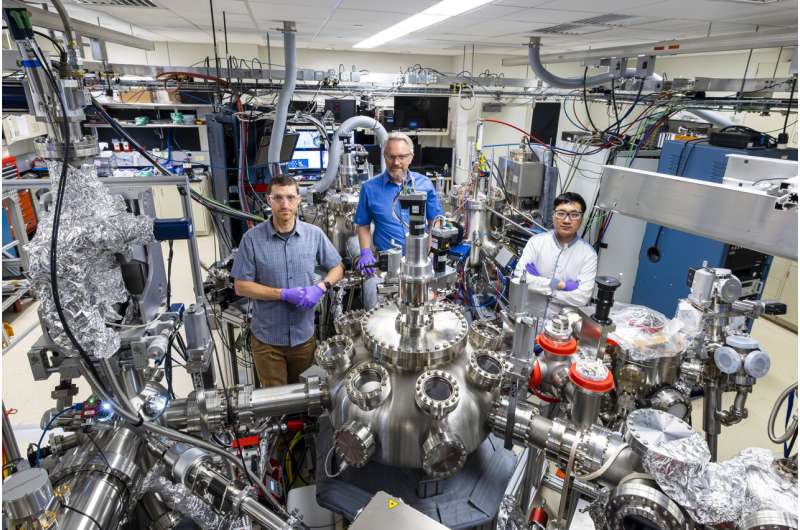
From left, Matthew Brahlek, Robert Moore, and Qiangsheng Lu foster topological superconducting materials for quantum figuring applications on the side of the Quantum Science Center settled at ORNL. Credit: Carlos Jones/ORNL, U.S. Dept. of Energy
Urgent difficulties remain. “We want to improve and better comprehend the materials at the nuclear level, which is basic to affirming and involving Majorana particles for applications,” Moore said. “The following stage will investigate conceivable Majorana particles utilizing a recently introduced ultralow-temperature filtering burrowing magnifying lens instrument at CNMS.”
He added, “Accomplishing a qubit in view of Majorana particles is one of the definitive objectives of the Quantum Science Center. The Majorana molecule in materials is in a particularly extraordinary state. Demonstrating that it exists will require both structure and testing a qubit-like gadget. It is an odd method for mulling over everything, except you need to make a qubit to demonstrate it is a qubit. We presently know how to control the materials to the level expected to get this going.”
More information: Robert G. Moore et al, Monolayer Superconductivity and Tunable Topological Electronic Structure at the Fe(Te,Se)/Bi2Te3 Interface, Advanced Materials (2023). DOI: 10.1002/adma.202210940