At the point when light goes through a material, it frequently acts in capricious ways. This peculiarity is the subject of a whole field of study called “nonlinear optics,” which is currently necessary for mechanical and logical advances from laser improvement and optical recurrence metrology to gravitational wave cosmology and quantum data science.
Moreover, ongoing years have seen nonlinear optics applied in optical sign handling, media communications, detecting, spectroscopy, light location, and more. This large number of applications includes the scaling down of gadgets that control light in nonlinear ways onto a little chip, empowering complex light cooperation on a chip scale.
Presently, a group of researchers at EPFL and the Maximum Board Establishment has brought nonlinear optical peculiarities into a transmission electron magnifying instrument (TEM), a kind of magnifying lens that involves electrons for imaging rather than light. The review was driven by Teacher Tobias J. Kippenberg at EPFL and Teacher Claus Ropers, Head of the Maximum Planck Organization for Multidisciplinary Sciences. It is currently distributed in science.
“We produced multiple nonlinear spatiotemporal light patterns in the microresonator using a continuous-wave laser. These light patterns interacted with an electron beam traveling through the photonic chip, leaving fingerprints in the electron spectrum.”
EPFL researcher Yujia Yang, who led the study.
At the core of the review are “Kerr solitons,” influxes of light that hold their shape and energy as they travel through a material, similar to an impeccably framed surf wave traversing the sea. This study utilized a specific sort of Kerr solitons called “dissipative,” which are steady, restricted beats of light that last many femtoseconds (a quadrillionth of a second) and structure suddenly in the microresonator. Dissipative Kerr solitons can likewise communicate with electrons, which made them urgent for this review.
The specialists framed dissipative Kerr solitons inside a photonic microresonator, a small chip that snares and circles light inside an intelligent hole, creating the ideal circumstances for these waves. “We created different nonlinear spatiotemporal light examples in the microresonator driven by a persistent wave laser,” makes sense of EPFL scientist Yujia Yang, who drove the review. “These light examples collaborated with a light emission passing by the photonic chip and left fingerprints in the electron range.”
In particular, the methodology showed the coupling between free electrons and dissipative Kerr solitons, which permitted the scientists to test soliton elements in the microresonator cavity and perform ultrafast adjustment of electron radiates.
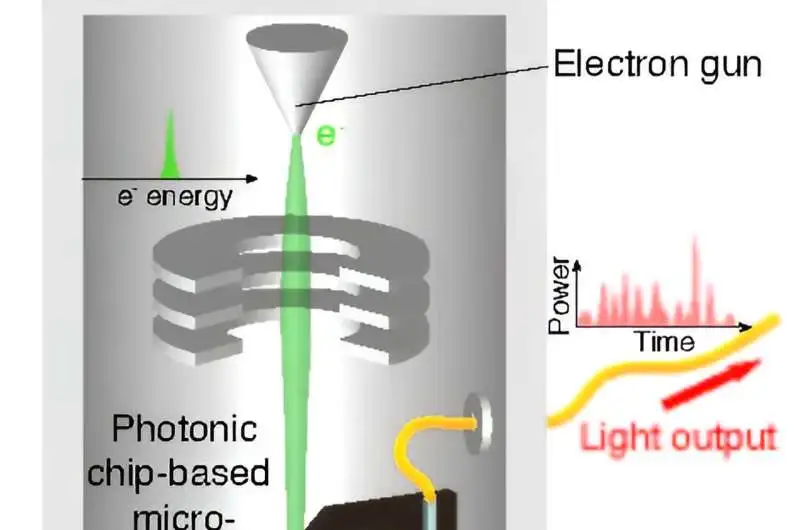
Schematic of the investigation. Nonlinear spatiotemporal light examples in a photonic chip-based microresonator tweak the range of light emission electrons in a transmission electron magnifying lens. Credit: Yang et al. DOI: 10.1126/science.adk2489
“Our capacity to create dissipative Kerr solitons [DKS] in a TEM expands the utilization of microresonator-base recurrence brushes to neglected domains,” says Kippenberg. “The electron-DKS cooperation could empower high-redundancy ultrafast electron microscopy and molecule gas pedals enabled by a little photonic chip.”
Ropers adds, “Our outcomes show electron microscopy could be a strong procedure for examining nonlinear optical elements at the nanoscale. This strategy is painless and ready to straightforwardly get to the intracavity field, key to understanding nonlinear optical physical science and creating nonlinear photonic gadgets.”
The photonic chips were manufactured in the focal point of MicroNanoTechnology (CMi) and the Foundation of Material Science cleanroom at EPFL. The examinations were directed at the Göttingen Ultrafast Transmission Electron Microscopy (UTEM) Lab.
More information: Yujia Yang et al, Free-electron interaction with nonlinear optical states in microresonators, Science (2024). DOI: 10.1126/science.adk2489. www.science.org/doi/10.1126/science.adk2489